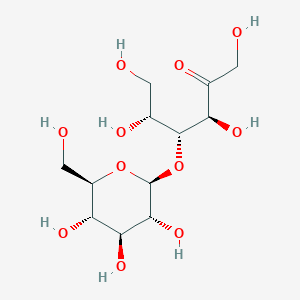
Cellobiulose
Overview
Description
Cellobiulose is a disaccharide composed of two glucose molecules linked by a β(1→4) glycosidic bond. It is a reducing sugar, meaning it has the ability to act as a reducing agent. This compound is derived from the partial hydrolysis of cellulose, a major component of plant cell walls. This compound is structurally similar to cellobiose, another disaccharide derived from cellulose, but differs in its specific glycosidic linkage.
Mechanism of Action
Target of Action
Cellobiulose, a disaccharide composed of two glucose units, primarily targets cellulase enzymes, which are responsible for the hydrolysis of cellulose . These enzymes include endoglucanases, which cut internal beta-1,4-glucosidic bonds, and exocellobiohydrolases . The primary role of these targets is to break down cellulose into simpler sugars, such as glucose, which can then be utilized by organisms for energy .
Mode of Action
This compound interacts with its targets (cellulase enzymes) through a process known as enzymatic hydrolysis . This process involves the cleavage of the beta-1,4-glycosidic bonds that hold the glucose units together in this compound . The cleavage of these bonds results in the formation of simpler sugars, such as glucose .
Biochemical Pathways
The biochemical pathway affected by this compound is the cellulose degradation pathway . In this pathway, this compound, through the action of cellulase enzymes, is broken down into simpler sugars, which can then enter glycolysis for energy production . The downstream effects of this pathway include the production of ATP, which is used as an energy source by cells .
Pharmacokinetics
It is known that this compound, like other sugars, is likely to be absorbed in the digestive tract and distributed throughout the body . Its metabolism would involve enzymatic hydrolysis to glucose, which can then be utilized for energy . Excretion would likely occur through the urinary system .
Result of Action
The molecular result of this compound’s action is the breakdown of its beta-1,4-glycosidic bonds, resulting in the formation of simpler sugars . On a cellular level, these simpler sugars can be utilized for energy production, contributing to various cellular processes .
Action Environment
The action of this compound is influenced by various environmental factors. For instance, the presence of specific enzymes (cellulases) is crucial for its breakdown . Additionally, factors such as pH and temperature can affect the activity of these enzymes and thus the rate of this compound breakdown . Furthermore, the presence of other compounds, such as LiCl/NMP, can influence the dissolution and subsequent breakdown of this compound .
Biochemical Analysis
Biochemical Properties
Cellobiulose metabolization involves high intracellular β-glucosidase activity . Unlike other fungal species that hydrolyze this compound extracellularly, certain strains transport this compound to the cytoplasm, where it is then hydrolyzed by high-affinity intracellular β-glucosidases .
Cellular Effects
The consumption of this compound can influence cellular function. For instance, during batch fermentation, the strain with the best performance consumed all the available this compound in the first 18 hours of the assay, producing 2.7 g L −1 of ethanol .
Molecular Mechanism
The molecular mechanism of this compound action involves its transport to the cytoplasm, where it is hydrolyzed by high-affinity intracellular β-glucosidases . This suggests a high-affinity hydrolytic system inside cells, with a Km of 12.4 mM .
Temporal Effects in Laboratory Settings
In laboratory settings, the effects of this compound can change over time. For instance, during batch fermentation, all the available this compound was consumed in the first 18 hours of the assay .
Transport and Distribution
This compound is transported to the cytoplasm of the cell, where it is then hydrolyzed by high-affinity intracellular β-glucosidases . This suggests that this compound is distributed within the cells where it is metabolized.
Subcellular Localization
The subcellular localization of this compound is within the cytoplasm of the cell, where it is hydrolyzed by high-affinity intracellular β-glucosidases . This suggests that this compound is directed to specific compartments within the cell for metabolization.
Preparation Methods
Synthetic Routes and Reaction Conditions: Cellobiulose can be synthesized through the enzymatic or acidic hydrolysis of cellulose. Enzymatic hydrolysis involves the use of cellulase enzymes, which break down cellulose into smaller sugar units, including this compound. Acidic hydrolysis, on the other hand, uses strong acids such as sulfuric acid to cleave the glycosidic bonds in cellulose, resulting in the formation of this compound.
Industrial Production Methods: In industrial settings, this compound is typically produced through the enzymatic hydrolysis of cellulose-rich materials such as cotton, jute, or paper. The process involves pretreating the cellulose material to increase its accessibility to enzymes, followed by the addition of cellulase enzymes to catalyze the hydrolysis reaction. The resulting mixture is then purified to isolate this compound.
Chemical Reactions Analysis
Types of Reactions: Cellobiulose undergoes various chemical reactions, including:
Hydrolysis: this compound can be hydrolyzed to produce glucose, a simple sugar with numerous applications in food and biofuel production.
Common Reagents and Conditions:
Oxidation: Common oxidizing agents include nitric acid and potassium permanganate.
Reduction: Reducing agents such as sodium borohydride or hydrogen gas in the presence of a catalyst are typically used.
Hydrolysis: Hydrolysis can be carried out using dilute acids or specific enzymes such as β-glucosidase.
Major Products:
Oxidation: this compound acid
Reduction: Cellobiitol
Hydrolysis: Glucose
Scientific Research Applications
It appears that the query is for the applications of "Cellobiulose," but the provided search results discuss "Cellobiose." Considering this, the following information will focus on the applications of cellobiose based on the provided search results.
Cellobiose is a disaccharide composed of two glucose molecules linked by a β-1,4-glycosidic bond . It can be produced from cellulose using specific bacterial enzymes and is hydrolyzed into glucose by cellobiosidase and cellulase . Cellobiose has garnered interest across various sectors, including food, feed, and cosmetics, spurring the need for cost-effective production methods .
Scientific Research Applications
Production and Bioconversion:
- One-pot synthesis: Cellobiose can be synthesized from sucrose using recombinant Pichia pastoris strains as reusable whole-cell biocatalysts . This process involves thermophilic sucrose phosphorylase from Bifidobacterium longum and cellobiose phosphorylase from Clostridium pastoris .
- Bioconversion cycles: Recombinant yeast cells can maintain a cellobiose titer > 80 g/L even after three consecutive cell-recycling one-pot bioconversion cycles, demonstrating high reusability as a whole-cell biocatalyst .
Metabolism and Engineering:
- Intracellular metabolism: Engineering industrial hosts like Escherichia coli and Saccharomyces cerevisiae for intracellular cellobiose metabolism can offer advantages in biomass technology . This approach can bypass the glucose/xylose mixed sugar co-utilization barrier, which is a significant challenge in using mixed sugars from renewable plant biomass .
- Ethanol production: Cellobiose-metabolizing yeast can eliminate the need for adding extra β-glucosidase to simultaneous saccharification and fermentation processes, favoring continuous fermentation for cellulosic ethanol production .
Health and Medical Applications:
- Prebiotic effects: Cellobiose has potential prebiotic effects, influencing bacterial composition and promoting immunomodulatory health effects . Studies in animals, such as horses and rabbits, have demonstrated these prebiotic effects .
- Colitis suppression: Cellobiose can suppress the development of dextran sulfate sodium (DSS) colitis in mice, reducing body weight loss and tissue edema .
- Safety and tolerability: A dose-escalation study demonstrated that 20 g of cellobiose is a tolerable dose for single use, with a recommendation of up to 15 g twice daily for repeated consumption .
Industrial Applications:
- Functional food additive: Cellobiose is recognized as an important functional food additive, driving the demand for cost-effective bioprocesses for its production .
- Biorefineries: Intracellular cellobiose metabolism is a promising alternative to secreted/surface-displayed β-glucosidases, allowing the use of cellobiose metabolism to bypass mixed sugar co-utilization barriers in lignocellulose-based biorefineries .
Table: Summary of Cellobiose Applications
Comparison with Similar Compounds
Cellobiose: Another disaccharide derived from cellulose, with a similar structure but different glycosidic linkage.
Maltose: A disaccharide composed of two glucose molecules linked by an α(1→4) glycosidic bond.
Lactose: A disaccharide composed of glucose and galactose linked by a β(1→4) glycosidic bond.
Uniqueness of Cellobiulose: this compound is unique in its specific β(1→4) glycosidic linkage, which distinguishes it from other disaccharides such as cellobiose and maltose. This unique structure influences its chemical reactivity and interactions with enzymes, making it a valuable compound for studying cellulose hydrolysis and developing new applications in various fields.
Biological Activity
Cellobiulose, a polysaccharide derived from cellulose, has garnered attention for its potential biological activities and applications in various fields, particularly in biochemistry and biotechnology. This article explores the biological activity of this compound, supported by research findings, case studies, and relevant data.
Overview of this compound
Chemical Structure and Properties
this compound is a linear polymer composed of repeating cellobiose units, which are disaccharides made up of two glucose molecules linked by a β-1,4-glycosidic bond. This structure contributes to its stability and resistance to enzymatic degradation compared to simple sugars.
Synthesis and Sources
this compound can be synthesized through the hydrolysis of cellulose or produced by certain microorganisms during fermentation processes. It serves as an intermediate in the conversion of biomass into biofuels and other chemicals.
1. Enzymatic Hydrolysis
Research indicates that this compound plays a crucial role in the enzymatic hydrolysis of cellulose. The cellulase system from Bacteroides cellulosolvens exhibits feedback inhibition by cellobiose, affecting cellulose solubilization. At concentrations above 2.6 g/L, cellobiose significantly inhibits cellulase activity, demonstrating its regulatory role in cellulose degradation processes .
2. Fermentation Processes
This compound is utilized as a substrate in fermentation processes by various microorganisms, including Clostridium acetobutylicum. Studies show that when this compound is used as a substrate, it supports microbial growth and metabolite production. For instance, fermentation experiments revealed that C. acetobutylicum could efficiently convert this compound into butanol and other solvents, highlighting its potential for biofuel production .
3. Antioxidant Activity
Emerging studies suggest that this compound possesses antioxidant properties. The oxidative degradation products of this compound have been shown to exhibit radical scavenging activity, which may contribute to its potential health benefits when used as a functional food ingredient .
Case Study 1: Hydrothermal Conversion
A study demonstrated that this compound acts as a key intermediate during the hydrothermal conversion of biomass into biofuels. The research highlighted the isomerization of cellobiose to glucose as a critical step in breaking glycosidic bonds during non-catalytic hydrothermal treatment .
Process Stage | Observations |
---|---|
Hydrolysis | Effective conversion of this compound to glucose |
Isomerization | Key role in glycosidic bond cleavage |
Yield Improvement | Enhanced biofuel production efficiency |
Case Study 2: Enzyme Characterization
The characterization of cellobiose dehydrogenases (CDHs) from various fungi has revealed their significant role in the oxidation of this compound. These enzymes demonstrate high substrate specificity for disaccharides like cellobiose and lactose, with catalytic efficiencies indicating their potential in biomass valorization .
Enzyme Source | Optimal pH | Catalytic Efficiency (M–1s–1) |
---|---|---|
Cellulomonas palmilytica | 6.0 | 2.05 x 10^5 |
Aspergillus niger | 5.5 | 9.06 x 10^4 |
Research Findings
Recent research has focused on optimizing the production and application of this compound in biorefinery processes. The integration of enzymatic pathways for efficient conversion of biomass into valuable products is a key area of investigation. For example, studies have shown that co-culturing microorganisms can enhance the degradation rates of this compound due to synergistic effects between different species .
Properties
IUPAC Name |
(3S,4R,5R)-1,3,5,6-tetrahydroxy-4-[(2S,3R,4S,5S,6R)-3,4,5-trihydroxy-6-(hydroxymethyl)oxan-2-yl]oxyhexan-2-one | |
---|---|---|
Details | Computed by LexiChem 2.6.6 (PubChem release 2019.06.18) | |
Source | PubChem | |
URL | https://pubchem.ncbi.nlm.nih.gov | |
Description | Data deposited in or computed by PubChem | |
InChI |
InChI=1S/C12H22O11/c13-1-4(16)7(18)11(5(17)2-14)23-12-10(21)9(20)8(19)6(3-15)22-12/h5-15,17-21H,1-3H2/t5-,6-,7-,8-,9+,10-,11-,12+/m1/s1 | |
Details | Computed by InChI 1.0.5 (PubChem release 2019.06.18) | |
Source | PubChem | |
URL | https://pubchem.ncbi.nlm.nih.gov | |
Description | Data deposited in or computed by PubChem | |
InChI Key |
PFCRQPBOOFTZGQ-WUYFHPBOSA-N | |
Details | Computed by InChI 1.0.5 (PubChem release 2019.06.18) | |
Source | PubChem | |
URL | https://pubchem.ncbi.nlm.nih.gov | |
Description | Data deposited in or computed by PubChem | |
Canonical SMILES |
C(C1C(C(C(C(O1)OC(C(CO)O)C(C(=O)CO)O)O)O)O)O | |
Details | Computed by OEChem 2.1.5 (PubChem release 2019.06.18) | |
Source | PubChem | |
URL | https://pubchem.ncbi.nlm.nih.gov | |
Description | Data deposited in or computed by PubChem | |
Isomeric SMILES |
C([C@@H]1[C@H]([C@@H]([C@H]([C@@H](O1)O[C@H]([C@@H](CO)O)[C@@H](C(=O)CO)O)O)O)O)O | |
Details | Computed by OEChem 2.1.5 (PubChem release 2019.06.18) | |
Source | PubChem | |
URL | https://pubchem.ncbi.nlm.nih.gov | |
Description | Data deposited in or computed by PubChem | |
Molecular Formula |
C12H22O11 | |
Details | Computed by PubChem 2.1 (PubChem release 2019.06.18) | |
Source | PubChem | |
URL | https://pubchem.ncbi.nlm.nih.gov | |
Description | Data deposited in or computed by PubChem | |
Molecular Weight |
342.30 g/mol | |
Details | Computed by PubChem 2.1 (PubChem release 2021.05.07) | |
Source | PubChem | |
URL | https://pubchem.ncbi.nlm.nih.gov | |
Description | Data deposited in or computed by PubChem | |
Disclaimer and Information on In-Vitro Research Products
Please be aware that all articles and product information presented on BenchChem are intended solely for informational purposes. The products available for purchase on BenchChem are specifically designed for in-vitro studies, which are conducted outside of living organisms. In-vitro studies, derived from the Latin term "in glass," involve experiments performed in controlled laboratory settings using cells or tissues. It is important to note that these products are not categorized as medicines or drugs, and they have not received approval from the FDA for the prevention, treatment, or cure of any medical condition, ailment, or disease. We must emphasize that any form of bodily introduction of these products into humans or animals is strictly prohibited by law. It is essential to adhere to these guidelines to ensure compliance with legal and ethical standards in research and experimentation.