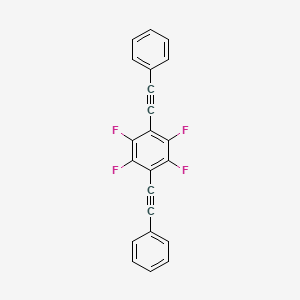
1,2,4,5-Tetrafluoro-3,6-bis(phenylethynyl)benzene
Overview
Description
1,2,4,5-Tetrafluoro-3,6-bis(phenylethynyl)benzene, also known as TFB, is an organofluorine compound. It has the molecular formula C22H10F4 and a molecular weight of 350.31 g/mol .
Molecular Structure Analysis
The molecular structure of 1,2,4,5-Tetrafluoro-3,6-bis(phenylethynyl)benzene consists of a benzene ring with two phenylethynyl groups attached at the 3 and 6 positions and four fluorine atoms attached at the 1,2,4,5 positions .Physical And Chemical Properties Analysis
The physical properties of 1,2,4,5-Tetrafluoro-3,6-bis(phenylethynyl)benzene include a melting point of 183-185 °C and a predicted boiling point of 444.6±45.0 °C. The compound has a predicted density of 1.34±0.1 g/cm3 .Scientific Research Applications
- Application : As a building block for organic semiconductors, this compound exhibits excellent charge transport properties. Researchers use it to create organic field-effect transistors (OFETs), organic light-emitting diodes (OLEDs), and organic photovoltaic cells (OPVs). Its high electron affinity and strong π-π stacking interactions contribute to efficient charge transport and light emission .
- Application : The unique electronic properties of 1,2,4,5-tetrafluoro-3,6-bis(phenylethynyl)benzene make it suitable for gas sensors. Researchers have explored its use in detecting volatile organic compounds (VOCs), such as environmental pollutants or industrial gases. Its sensitivity to specific analytes can lead to highly selective sensors .
- Application : Incorporating this compound into polymer matrices enhances their mechanical and thermal properties. It improves the stability and performance of composite materials, making them suitable for aerospace, automotive, and structural applications. Researchers investigate its role in reinforcing polymers and composites .
- Application : Researchers study its excited-state behavior, fluorescence, and phosphorescence properties. Understanding its photophysical processes aids in designing luminescent materials, sensors, and imaging agents. Its rigid structure and fluorine atoms contribute to efficient energy transfer and emission .
- Application : Researchers explore its potential as an electrocatalyst for various reactions, such as oxygen reduction and hydrogen evolution. Its π-conjugated system and electron-rich nature enhance catalytic activity. Investigating its stability and selectivity is crucial for sustainable energy applications .
- Application : Chemists use 1,2,4,5-tetrafluoro-3,6-bis(phenylethynyl)benzene as a derivatizing agent in gas chromatography-electron-capture negative ion mass spectrometry (GC-ECNI-MS). It helps improve the detection and identification of analytes by enhancing their volatility and ionization efficiency .
Organic Electronics and Optoelectronics
Chemical Sensors
Materials Science
Photochemistry and Photophysics
Electrochemical Catalysis
Derivatizing Agent in Mass Spectrometry
properties
IUPAC Name |
1,2,4,5-tetrafluoro-3,6-bis(2-phenylethynyl)benzene | |
---|---|---|
Details | Computed by Lexichem TK 2.7.0 (PubChem release 2021.05.07) | |
Source | PubChem | |
URL | https://pubchem.ncbi.nlm.nih.gov | |
Description | Data deposited in or computed by PubChem | |
InChI |
InChI=1S/C22H10F4/c23-19-17(13-11-15-7-3-1-4-8-15)20(24)22(26)18(21(19)25)14-12-16-9-5-2-6-10-16/h1-10H | |
Details | Computed by InChI 1.0.6 (PubChem release 2021.05.07) | |
Source | PubChem | |
URL | https://pubchem.ncbi.nlm.nih.gov | |
Description | Data deposited in or computed by PubChem | |
InChI Key |
ZNPXKSNJBLIZIP-UHFFFAOYSA-N | |
Details | Computed by InChI 1.0.6 (PubChem release 2021.05.07) | |
Source | PubChem | |
URL | https://pubchem.ncbi.nlm.nih.gov | |
Description | Data deposited in or computed by PubChem | |
Canonical SMILES |
C1=CC=C(C=C1)C#CC2=C(C(=C(C(=C2F)F)C#CC3=CC=CC=C3)F)F | |
Details | Computed by OEChem 2.3.0 (PubChem release 2021.05.07) | |
Source | PubChem | |
URL | https://pubchem.ncbi.nlm.nih.gov | |
Description | Data deposited in or computed by PubChem | |
Molecular Formula |
C22H10F4 | |
Details | Computed by PubChem 2.1 (PubChem release 2021.05.07) | |
Source | PubChem | |
URL | https://pubchem.ncbi.nlm.nih.gov | |
Description | Data deposited in or computed by PubChem | |
Molecular Weight |
350.3 g/mol | |
Details | Computed by PubChem 2.1 (PubChem release 2021.05.07) | |
Source | PubChem | |
URL | https://pubchem.ncbi.nlm.nih.gov | |
Description | Data deposited in or computed by PubChem | |
Product Name |
1,2,4,5-Tetrafluoro-3,6-bis(2-phenylethynyl)benzene |
Retrosynthesis Analysis
AI-Powered Synthesis Planning: Our tool employs the Template_relevance Pistachio, Template_relevance Bkms_metabolic, Template_relevance Pistachio_ringbreaker, Template_relevance Reaxys, Template_relevance Reaxys_biocatalysis model, leveraging a vast database of chemical reactions to predict feasible synthetic routes.
One-Step Synthesis Focus: Specifically designed for one-step synthesis, it provides concise and direct routes for your target compounds, streamlining the synthesis process.
Accurate Predictions: Utilizing the extensive PISTACHIO, BKMS_METABOLIC, PISTACHIO_RINGBREAKER, REAXYS, REAXYS_BIOCATALYSIS database, our tool offers high-accuracy predictions, reflecting the latest in chemical research and data.
Strategy Settings
Precursor scoring | Relevance Heuristic |
---|---|
Min. plausibility | 0.01 |
Model | Template_relevance |
Template Set | Pistachio/Bkms_metabolic/Pistachio_ringbreaker/Reaxys/Reaxys_biocatalysis |
Top-N result to add to graph | 6 |
Feasible Synthetic Routes
Disclaimer and Information on In-Vitro Research Products
Please be aware that all articles and product information presented on BenchChem are intended solely for informational purposes. The products available for purchase on BenchChem are specifically designed for in-vitro studies, which are conducted outside of living organisms. In-vitro studies, derived from the Latin term "in glass," involve experiments performed in controlled laboratory settings using cells or tissues. It is important to note that these products are not categorized as medicines or drugs, and they have not received approval from the FDA for the prevention, treatment, or cure of any medical condition, ailment, or disease. We must emphasize that any form of bodily introduction of these products into humans or animals is strictly prohibited by law. It is essential to adhere to these guidelines to ensure compliance with legal and ethical standards in research and experimentation.