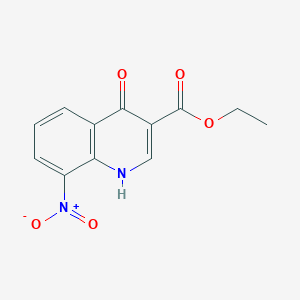
Ethyl 4-hydroxy-8-nitroquinoline-3-carboxylate
Overview
Description
Ethyl 4-hydroxy-8-nitroquinoline-3-carboxylate is a chemical compound with the molecular formula C₁₂H₁₀N₂O₅. It is a derivative of quinoline, a heterocyclic aromatic organic compound. This compound is known for its diverse applications in scientific research, particularly in the fields of chemistry, biology, and medicine .
Preparation Methods
Synthetic Routes and Reaction Conditions
The synthesis of Ethyl 4-hydroxy-8-nitroquinoline-3-carboxylate typically involves the nitration of ethyl 4-hydroxyquinoline-3-carboxylate. The reaction is carried out under controlled conditions using nitric acid and sulfuric acid as nitrating agents. The reaction mixture is maintained at a low temperature to prevent over-nitration and to ensure the selective formation of the desired product .
Industrial Production Methods
Industrial production of this compound follows similar synthetic routes but on a larger scale. The process involves the use of large reactors and precise control of reaction parameters to ensure high yield and purity of the product. The compound is then purified through recrystallization or chromatography techniques .
Chemical Reactions Analysis
Types of Reactions
Ethyl 4-hydroxy-8-nitroquinoline-3-carboxylate undergoes various chemical reactions, including:
Oxidation: The compound can be oxidized to form quinoline derivatives with different functional groups.
Reduction: Reduction of the nitro group can yield amino derivatives.
Substitution: The hydroxyl and nitro groups can participate in substitution reactions to form various derivatives
Common Reagents and Conditions
Oxidation: Common oxidizing agents include potassium permanganate and chromium trioxide.
Reduction: Reducing agents such as hydrogen gas in the presence of a palladium catalyst or sodium borohydride are used.
Substitution: Reagents like alkyl halides or acyl chlorides are used under basic or acidic conditions
Major Products Formed
Oxidation: Quinoline derivatives with carboxylic acid or ketone functional groups.
Reduction: Aminoquinoline derivatives.
Substitution: Alkylated or acylated quinoline derivatives
Scientific Research Applications
Ethyl 4-hydroxy-8-nitroquinoline-3-carboxylate has a wide range of applications in scientific research:
Chemistry: Used as a building block for the synthesis of complex organic molecules.
Biology: Investigated for its potential as an antimicrobial and antiviral agent.
Medicine: Studied for its potential therapeutic effects in treating various diseases, including cancer and infectious diseases.
Industry: Utilized in the development of dyes, pigments, and other industrial chemicals.
Mechanism of Action
The mechanism of action of Ethyl 4-hydroxy-8-nitroquinoline-3-carboxylate involves its interaction with specific molecular targets and pathways. The compound can inhibit the activity of certain enzymes, disrupt cellular processes, and induce oxidative stress in target cells. These effects are mediated through the formation of reactive oxygen species and the modulation of signaling pathways .
Comparison with Similar Compounds
Ethyl 4-hydroxy-8-nitroquinoline-3-carboxylate can be compared with other similar compounds, such as:
- Ethyl 4-hydroxy-6-nitroquinoline-3-carboxylate
- Ethyl 2-hydroxy-6-methylpyridine-4-carboxylate
- 4-Hydroxy-6-nitroquinoline-3-carboxylic acid
- 2-Ethyl-4-methyl-8-nitroquinoline
These compounds share similar structural features but differ in their functional groups and specific applications. This compound is unique due to its specific substitution pattern, which imparts distinct chemical and biological properties .
Properties
IUPAC Name |
ethyl 8-nitro-4-oxo-1H-quinoline-3-carboxylate | |
---|---|---|
Details | Computed by LexiChem 2.6.6 (PubChem release 2019.06.18) | |
Source | PubChem | |
URL | https://pubchem.ncbi.nlm.nih.gov | |
Description | Data deposited in or computed by PubChem | |
InChI |
InChI=1S/C12H10N2O5/c1-2-19-12(16)8-6-13-10-7(11(8)15)4-3-5-9(10)14(17)18/h3-6H,2H2,1H3,(H,13,15) | |
Details | Computed by InChI 1.0.5 (PubChem release 2019.06.18) | |
Source | PubChem | |
URL | https://pubchem.ncbi.nlm.nih.gov | |
Description | Data deposited in or computed by PubChem | |
InChI Key |
ZTAWASGMGQOLHO-UHFFFAOYSA-N | |
Details | Computed by InChI 1.0.5 (PubChem release 2019.06.18) | |
Source | PubChem | |
URL | https://pubchem.ncbi.nlm.nih.gov | |
Description | Data deposited in or computed by PubChem | |
Canonical SMILES |
CCOC(=O)C1=CNC2=C(C1=O)C=CC=C2[N+](=O)[O-] | |
Details | Computed by OEChem 2.1.5 (PubChem release 2019.06.18) | |
Source | PubChem | |
URL | https://pubchem.ncbi.nlm.nih.gov | |
Description | Data deposited in or computed by PubChem | |
Molecular Formula |
C12H10N2O5 | |
Details | Computed by PubChem 2.1 (PubChem release 2019.06.18) | |
Source | PubChem | |
URL | https://pubchem.ncbi.nlm.nih.gov | |
Description | Data deposited in or computed by PubChem | |
DSSTOX Substance ID |
DTXSID60903624 | |
Record name | NoName_4327 | |
Source | EPA DSSTox | |
URL | https://comptox.epa.gov/dashboard/DTXSID60903624 | |
Description | DSSTox provides a high quality public chemistry resource for supporting improved predictive toxicology. | |
Molecular Weight |
262.22 g/mol | |
Details | Computed by PubChem 2.1 (PubChem release 2021.05.07) | |
Source | PubChem | |
URL | https://pubchem.ncbi.nlm.nih.gov | |
Description | Data deposited in or computed by PubChem | |
Synthesis routes and methods
Procedure details
Retrosynthesis Analysis
AI-Powered Synthesis Planning: Our tool employs the Template_relevance Pistachio, Template_relevance Bkms_metabolic, Template_relevance Pistachio_ringbreaker, Template_relevance Reaxys, Template_relevance Reaxys_biocatalysis model, leveraging a vast database of chemical reactions to predict feasible synthetic routes.
One-Step Synthesis Focus: Specifically designed for one-step synthesis, it provides concise and direct routes for your target compounds, streamlining the synthesis process.
Accurate Predictions: Utilizing the extensive PISTACHIO, BKMS_METABOLIC, PISTACHIO_RINGBREAKER, REAXYS, REAXYS_BIOCATALYSIS database, our tool offers high-accuracy predictions, reflecting the latest in chemical research and data.
Strategy Settings
Precursor scoring | Relevance Heuristic |
---|---|
Min. plausibility | 0.01 |
Model | Template_relevance |
Template Set | Pistachio/Bkms_metabolic/Pistachio_ringbreaker/Reaxys/Reaxys_biocatalysis |
Top-N result to add to graph | 6 |
Feasible Synthetic Routes
Disclaimer and Information on In-Vitro Research Products
Please be aware that all articles and product information presented on BenchChem are intended solely for informational purposes. The products available for purchase on BenchChem are specifically designed for in-vitro studies, which are conducted outside of living organisms. In-vitro studies, derived from the Latin term "in glass," involve experiments performed in controlled laboratory settings using cells or tissues. It is important to note that these products are not categorized as medicines or drugs, and they have not received approval from the FDA for the prevention, treatment, or cure of any medical condition, ailment, or disease. We must emphasize that any form of bodily introduction of these products into humans or animals is strictly prohibited by law. It is essential to adhere to these guidelines to ensure compliance with legal and ethical standards in research and experimentation.