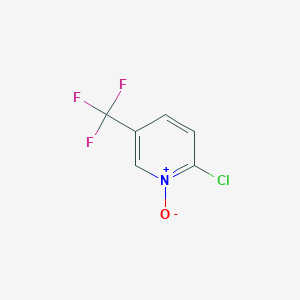
2-Chloro-5-(trifluoromethyl)pyridine 1-oxide
Overview
Description
2-Chloro-5-(trifluoromethyl)pyridine 1-oxide is an organic compound with the molecular formula C6H3ClF3NO. It is a derivative of pyridine, characterized by the presence of a chlorine atom and a trifluoromethyl group attached to the pyridine ring, along with an oxide group. This compound is known for its applications in various fields, including organic synthesis and pharmaceuticals.
Mechanism of Action
Target of Action
The primary target of 2-Chloro-5-(trifluoromethyl)pyridine 1-oxide is the respiratory system . This compound interacts with the respiratory system, causing changes in its function and potentially leading to respiratory irritation .
Mode of Action
It is known that the compound can causegenetic defects , suggesting that it may interact with DNA or other genetic material. It is also known to cause skin and eye irritation, indicating that it interacts with these tissues in a harmful way .
Biochemical Pathways
Given its effects on the respiratory system and its potential to cause genetic defects, it is likely that it impacts pathways related tocellular respiration and DNA repair .
Pharmacokinetics
Its harmful effects suggest that it is bioavailable and can reach its target tissues after exposure .
Result of Action
The result of the action of this compound includes respiratory irritation , skin irritation , eye irritation , and potentially genetic defects . These effects indicate that the compound has a significant impact at the molecular and cellular levels.
Action Environment
The action of this compound can be influenced by various environmental factors. For instance, its stability and efficacy may be affected by factors such as temperature, pH, and the presence of other chemicals . .
Preparation Methods
Synthetic Routes and Reaction Conditions: The synthesis of 2-Chloro-5-(trifluoromethyl)pyridine 1-oxide can be achieved through several methods. One common approach involves the reaction of 2-Chloro-5-(trifluoromethyl)pyridine with an oxidizing agent. For instance, the oxidation can be carried out using hydrogen peroxide or peracids under controlled conditions to introduce the oxide group .
Industrial Production Methods: Industrial production of this compound typically involves large-scale oxidation processes. The starting material, 2-Chloro-5-(trifluoromethyl)pyridine, is subjected to oxidation in the presence of suitable catalysts and oxidizing agents. The reaction conditions, such as temperature, pressure, and solvent, are optimized to achieve high yields and purity .
Chemical Reactions Analysis
Types of Reactions: 2-Chloro-5-(trifluoromethyl)pyridine 1-oxide undergoes various chemical reactions, including:
Oxidation: Further oxidation can lead to the formation of more oxidized derivatives.
Reduction: Reduction reactions can convert the oxide group back to the parent pyridine compound.
Substitution: The chlorine atom can be substituted with other functional groups through nucleophilic substitution reactions.
Common Reagents and Conditions:
Oxidation: Hydrogen peroxide, peracids.
Reduction: Metal hydrides, catalytic hydrogenation.
Substitution: Nucleophiles such as amines, thiols, and alkoxides.
Major Products: The major products formed from these reactions depend on the specific reagents and conditions used. For example, nucleophilic substitution can yield various substituted pyridine derivatives .
Scientific Research Applications
2-Chloro-5-(trifluoromethyl)pyridine 1-oxide has a wide range of applications in scientific research:
Chemistry: It is used as a building block in organic synthesis, enabling the creation of more complex molecules.
Biology: The compound is studied for its potential biological activities, including antimicrobial and anticancer properties.
Medicine: It serves as an intermediate in the synthesis of pharmaceutical compounds.
Industry: The compound is used in the production of agrochemicals, such as herbicides and insecticides
Comparison with Similar Compounds
- 2-Chloro-4-(trifluoromethyl)pyridine
- 2-Bromo-5-(trifluoromethyl)pyridine
- 2-Chloro-5-iodopyridine
- 2-Hydroxy-5-(trifluoromethyl)pyridine
Comparison: Compared to its analogs, 2-Chloro-5-(trifluoromethyl)pyridine 1-oxide is unique due to the presence of the oxide group, which imparts distinct chemical reactivity and biological activity. The trifluoromethyl group enhances its stability and lipophilicity, making it a valuable intermediate in various chemical and pharmaceutical applications .
Properties
IUPAC Name |
2-chloro-1-oxido-5-(trifluoromethyl)pyridin-1-ium | |
---|---|---|
Details | Computed by LexiChem 2.6.6 (PubChem release 2019.06.18) | |
Source | PubChem | |
URL | https://pubchem.ncbi.nlm.nih.gov | |
Description | Data deposited in or computed by PubChem | |
InChI |
InChI=1S/C6H3ClF3NO/c7-5-2-1-4(3-11(5)12)6(8,9)10/h1-3H | |
Details | Computed by InChI 1.0.5 (PubChem release 2019.06.18) | |
Source | PubChem | |
URL | https://pubchem.ncbi.nlm.nih.gov | |
Description | Data deposited in or computed by PubChem | |
InChI Key |
BQICMLHZXPNIBU-UHFFFAOYSA-N | |
Details | Computed by InChI 1.0.5 (PubChem release 2019.06.18) | |
Source | PubChem | |
URL | https://pubchem.ncbi.nlm.nih.gov | |
Description | Data deposited in or computed by PubChem | |
Canonical SMILES |
C1=CC(=[N+](C=C1C(F)(F)F)[O-])Cl | |
Details | Computed by OEChem 2.1.5 (PubChem release 2019.06.18) | |
Source | PubChem | |
URL | https://pubchem.ncbi.nlm.nih.gov | |
Description | Data deposited in or computed by PubChem | |
Molecular Formula |
C6H3ClF3NO | |
Details | Computed by PubChem 2.1 (PubChem release 2019.06.18) | |
Source | PubChem | |
URL | https://pubchem.ncbi.nlm.nih.gov | |
Description | Data deposited in or computed by PubChem | |
Molecular Weight |
197.54 g/mol | |
Details | Computed by PubChem 2.1 (PubChem release 2021.05.07) | |
Source | PubChem | |
URL | https://pubchem.ncbi.nlm.nih.gov | |
Description | Data deposited in or computed by PubChem | |
Synthesis routes and methods I
Procedure details
Synthesis routes and methods II
Procedure details
Synthesis routes and methods III
Procedure details
Synthesis routes and methods IV
Procedure details
Retrosynthesis Analysis
AI-Powered Synthesis Planning: Our tool employs the Template_relevance Pistachio, Template_relevance Bkms_metabolic, Template_relevance Pistachio_ringbreaker, Template_relevance Reaxys, Template_relevance Reaxys_biocatalysis model, leveraging a vast database of chemical reactions to predict feasible synthetic routes.
One-Step Synthesis Focus: Specifically designed for one-step synthesis, it provides concise and direct routes for your target compounds, streamlining the synthesis process.
Accurate Predictions: Utilizing the extensive PISTACHIO, BKMS_METABOLIC, PISTACHIO_RINGBREAKER, REAXYS, REAXYS_BIOCATALYSIS database, our tool offers high-accuracy predictions, reflecting the latest in chemical research and data.
Strategy Settings
Precursor scoring | Relevance Heuristic |
---|---|
Min. plausibility | 0.01 |
Model | Template_relevance |
Template Set | Pistachio/Bkms_metabolic/Pistachio_ringbreaker/Reaxys/Reaxys_biocatalysis |
Top-N result to add to graph | 6 |
Feasible Synthetic Routes
Disclaimer and Information on In-Vitro Research Products
Please be aware that all articles and product information presented on BenchChem are intended solely for informational purposes. The products available for purchase on BenchChem are specifically designed for in-vitro studies, which are conducted outside of living organisms. In-vitro studies, derived from the Latin term "in glass," involve experiments performed in controlled laboratory settings using cells or tissues. It is important to note that these products are not categorized as medicines or drugs, and they have not received approval from the FDA for the prevention, treatment, or cure of any medical condition, ailment, or disease. We must emphasize that any form of bodily introduction of these products into humans or animals is strictly prohibited by law. It is essential to adhere to these guidelines to ensure compliance with legal and ethical standards in research and experimentation.