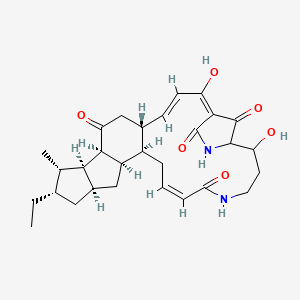
Xanthobaccin A
Overview
Description
Xanthobaccin A is a natural antibiotic compound produced by the bacterium Stenotrophomonas sp. strain SB-K88. It is known for its potent antifungal properties, particularly in suppressing damping-off disease in sugar beet seedlings caused by soilborne pathogens such as Pythium spp. and Rhizoctonia solani .
Mechanism of Action
Target of Action
Xanthobaccin A, a potent antifungal compound, is primarily targeted towards soilborne plant pathogens, including Rhizoctonia solani , Pythium ultimum , and Aphanomyces cochlioides . These pathogens are known to cause damping-off disease in sugar beet seedlings . The compound’s primary role is to suppress this disease, thereby promoting the health and growth of the plants .
Mode of Action
The mode of action of this compound is quite unique. It has been identified as a metallophore , a compound that can bind and transport metal ions . This compound, along with other tetramates, has been found to reduce ferric (Fe III) into ferrous (Fe II) iron . This reduction triggers the Fenton chemistry reaction , a process that can produce hydroxyl radicals . These radicals are highly reactive and can cause damage to various cellular components, thereby exhibiting antibacterial activity .
Biochemical Pathways
It is known that the compound is produced by the bacteriumStenotrophomonas sp. strain SB-K88 . This bacterium is a rhizobacterium of sugar beet and is known to suppress damping-off disease . The production of this compound in culture media has been linked to the disease suppression activities of the strain .
Pharmacokinetics
It has been observed that the compound can be produced in the rhizosphere of seedlings when the strain sb-k88 is applied to sugar beet seeds . This suggests that the compound may have good bioavailability in the plant’s immediate environment.
Result of Action
The primary result of this compound’s action is the suppression of damping-off disease in sugar beet seedlings . By reducing the activity of soilborne plant pathogens, the compound promotes the health and growth of the plants . Additionally, the compound’s ability to trigger Fenton chemistry and produce hydroxyl radicals suggests that it may have broad antimicrobial activity .
Action Environment
The action of this compound is influenced by the environmental conditions in the rhizosphere of the plants. The compound is produced by the bacterium Stenotrophomonas sp. strain SB-K88, which thrives in this environment . The production of this compound and its subsequent effects on plant pathogens are likely to be influenced by factors such as soil composition, moisture levels, and the presence of other microorganisms.
Biochemical Analysis
Biochemical Properties
Xanthobaccin A plays a crucial role in biochemical reactions, particularly in its interactions with various enzymes and proteins. It has been shown to inhibit the growth of several fungal pathogens by interfering with their cellular processes. This compound interacts with enzymes involved in cell wall synthesis, leading to the disruption of fungal cell walls and subsequent cell lysis . Additionally, it has been observed to bind to bacterial ribosomes, inhibiting protein synthesis and thereby exerting its antibacterial effects .
Cellular Effects
This compound exerts significant effects on various types of cells and cellular processes. In fungal cells, it disrupts cell wall integrity, leading to cell lysis and death. In bacterial cells, this compound inhibits protein synthesis by binding to ribosomes, which hampers bacterial growth and proliferation . Furthermore, this compound has been shown to influence cell signaling pathways and gene expression, particularly those involved in stress responses and metabolic processes .
Molecular Mechanism
The molecular mechanism of this compound involves its binding interactions with key biomolecules. It binds to fungal cell wall synthesis enzymes, inhibiting their activity and leading to cell wall disruption . In bacterial cells, this compound binds to ribosomal subunits, preventing the translation of essential proteins . Additionally, it has been suggested that this compound may induce oxidative stress in target cells by generating reactive oxygen species (ROS), further contributing to its antimicrobial effects .
Temporal Effects in Laboratory Settings
In laboratory settings, the effects of this compound have been observed to change over time. The compound exhibits stability under various conditions, maintaining its antifungal and antibacterial activities for extended periods . Prolonged exposure to this compound can lead to the development of resistance in some microbial populations, necessitating careful management of its use . Long-term studies have shown that this compound can have lasting effects on cellular function, including alterations in gene expression and metabolic activity .
Dosage Effects in Animal Models
The effects of this compound vary with different dosages in animal models. At lower doses, this compound effectively inhibits microbial growth without causing significant toxicity . At higher doses, it can induce adverse effects, including cytotoxicity and oxidative stress . Threshold effects have been observed, where a minimum concentration of this compound is required to achieve its antimicrobial effects . Careful dosage optimization is essential to maximize its therapeutic potential while minimizing adverse effects .
Metabolic Pathways
This compound is involved in several metabolic pathways, particularly those related to its biosynthesis and degradation. The compound is synthesized through a complex pathway involving polyketide synthases and non-ribosomal peptide synthetases . It interacts with various enzymes and cofactors during its biosynthesis, including acyltransferases and dehydrogenases . Additionally, this compound can influence metabolic flux and metabolite levels in target cells, further contributing to its antimicrobial effects .
Transport and Distribution
Within cells and tissues, this compound is transported and distributed through specific transporters and binding proteins. It has been observed to accumulate in the rhizosphere of plants treated with Stenotrophomonas sp. strain SB-K88, where it exerts its antifungal effects . The compound’s localization and accumulation are influenced by its interactions with transport proteins and cellular membranes . Understanding the transport and distribution mechanisms of this compound is crucial for optimizing its application in agricultural and medical settings .
Subcellular Localization
This compound exhibits specific subcellular localization patterns that are essential for its activity and function. In fungal cells, it localizes to the cell wall, where it disrupts cell wall synthesis and integrity . In bacterial cells, this compound is found in the cytoplasm, where it binds to ribosomes and inhibits protein synthesis . The compound’s subcellular localization is directed by targeting signals and post-translational modifications that ensure its delivery to the appropriate cellular compartments .
Preparation Methods
Synthetic Routes and Reaction Conditions
Xanthobaccin A is typically isolated from the culture fluid of Stenotrophomonas sp. strain SB-K88. The isolation process involves scale-up fermentation, followed by extraction and purification using techniques such as mass spectrometry and nuclear magnetic resonance spectroscopy .
Industrial Production Methods
Currently, there are no well-documented industrial production methods for this compound. The compound is primarily obtained through microbial fermentation processes involving the cultivation of Stenotrophomonas sp. strain SB-K88 under controlled conditions .
Chemical Reactions Analysis
Types of Reactions
Xanthobaccin A undergoes various chemical reactions, including oxidation and reduction. It has been shown to reduce ferric iron (Fe(III)) to ferrous iron (Fe(II)), which triggers the Fenton chemistry reaction .
Common Reagents and Conditions
The reduction of ferric iron by this compound can be influenced by the presence of radical scavengers such as vitamin C, which can reduce the antibacterial activity of the compound .
Major Products Formed
The major products formed from the reactions involving this compound include ferrous iron and other intermediates that participate in the Fenton chemistry reaction .
Scientific Research Applications
Xanthobaccin A has several scientific research applications:
Agriculture: It is used as a biocontrol agent to suppress damping-off disease in sugar beet seedlings.
Microbiology: This compound exhibits antibacterial activity against Bacillus subtilis and other bacterial strains.
Biochemistry: The compound acts as a metallophore, chelating metal ions and participating in redox reactions.
Comparison with Similar Compounds
Xanthobaccin A is part of the polycyclic tetramate macrolactams family, which includes other compounds such as equisetin and ikarugamycin. These compounds share similar antibacterial properties and mechanisms of action. this compound is unique in its specific application as a biocontrol agent in agriculture .
List of Similar Compounds
- Equisetin
- Ikarugamycin
Biological Activity
Xanthobaccin A is a bioactive compound produced by the bacterium Stenotrophomonas sp. strain SB-K88, known for its significant antimicrobial properties, particularly against plant pathogens. This article delves into the biological activities associated with this compound, highlighting its mechanisms of action, efficacy against various microorganisms, and potential applications in agriculture and medicine.
Chemical Structure and Properties
This compound is classified as a tetramic acid-containing macrolactam. Its unique structure features a 5,5,6-tricyclic skeleton and a tetramic acid moiety, which contribute to its biological activity. The compound has been isolated and characterized through advanced techniques such as mass spectrometry and nuclear magnetic resonance (NMR) spectroscopy .
This compound exhibits its antimicrobial effects primarily through:
- Iron Chelation : The compound can chelate ferric iron and reduce it to ferrous iron, initiating Fenton chemistry, which generates reactive oxygen species (ROS) that are toxic to microorganisms .
- Direct Antifungal Activity : It has shown potent inhibitory effects against various fungi responsible for damping-off diseases in plants, particularly those caused by Pythium species .
Efficacy Against Pathogens
This compound has demonstrated effectiveness against:
- Bacteria : It shows antibacterial activity against Bacillus subtilis and other Gram-positive bacteria .
- Fungi : In vitro studies have confirmed its antifungal properties against pathogens like Pythium spp., leading to significant suppression of damping-off disease in sugar beet .
Suppression of Damping-Off Disease
A notable study involved the application of this compound in agricultural settings. The compound was directly applied to sugar beet seeds, resulting in a marked reduction in damping-off disease incidence. The study quantified the production of this compound in the rhizosphere, confirming its role in disease suppression. The results are summarized in the table below:
Treatment | Disease Suppression (%) | In Vitro Production (mg/L) | Concentration in Rhizosphere (μg/plant) |
---|---|---|---|
Control | 12.1 ± 6.0 | 0 | 0 |
SB-K88 | 35.8 ± 6.7 | 4.1 | 16.7 |
SB-K88-a56 | 29.3 ± 7.3 | 4.9 | 4.7 |
SB-K88-a120 | 32.8 ± 7.6 | 5.3 | 0.7 |
This data illustrates the correlation between this compound production and effective disease suppression .
Agricultural Use
Due to its antifungal properties, this compound holds promise as a natural pesticide alternative, particularly for crops susceptible to damping-off diseases. Its application could lead to reduced reliance on synthetic fungicides, promoting sustainable agricultural practices.
Potential Medical Applications
Research into this compound's antimicrobial properties suggests potential applications in developing new antibiotics or antifungal agents for clinical use, especially given the rising concern over antibiotic resistance.
Properties
IUPAC Name |
(1E,3E,5R,8S,9S,10S,11S,13R,15R,16R,18Z)-11-ethyl-2,24-dihydroxy-10-methyl-21,26-diazapentacyclo[23.2.1.05,16.08,15.09,13]octacosa-1,3,18-triene-7,20,27,28-tetrone | |
---|---|---|
Details | Computed by Lexichem TK 2.7.0 (PubChem release 2021.05.07) | |
Source | PubChem | |
URL | https://pubchem.ncbi.nlm.nih.gov | |
Description | Data deposited in or computed by PubChem | |
InChI |
InChI=1S/C29H38N2O6/c1-3-15-11-17-12-19-18-5-4-6-23(35)30-10-9-21(33)27-28(36)26(29(37)31-27)20(32)8-7-16(18)13-22(34)25(19)24(17)14(15)2/h4,6-8,14-19,21,24-25,27,32-33H,3,5,9-13H2,1-2H3,(H,30,35)(H,31,37)/b6-4-,8-7+,26-20+/t14-,15-,16-,17+,18+,19+,21?,24+,25+,27?/m0/s1 | |
Details | Computed by InChI 1.0.6 (PubChem release 2021.05.07) | |
Source | PubChem | |
URL | https://pubchem.ncbi.nlm.nih.gov | |
Description | Data deposited in or computed by PubChem | |
InChI Key |
GXFBXYRIFPTSTH-IELMVSCISA-N | |
Details | Computed by InChI 1.0.6 (PubChem release 2021.05.07) | |
Source | PubChem | |
URL | https://pubchem.ncbi.nlm.nih.gov | |
Description | Data deposited in or computed by PubChem | |
Canonical SMILES |
CCC1CC2CC3C4CC=CC(=O)NCCC(C5C(=O)C(=C(C=CC4CC(=O)C3C2C1C)O)C(=O)N5)O | |
Details | Computed by OEChem 2.3.0 (PubChem release 2021.05.07) | |
Source | PubChem | |
URL | https://pubchem.ncbi.nlm.nih.gov | |
Description | Data deposited in or computed by PubChem | |
Isomeric SMILES |
CC[C@H]1C[C@@H]2C[C@@H]3[C@@H]4C/C=C\C(=O)NCCC(C5C(=O)/C(=C(/C=C/[C@H]4CC(=O)[C@@H]3[C@@H]2[C@H]1C)\O)/C(=O)N5)O | |
Details | Computed by OEChem 2.3.0 (PubChem release 2021.05.07) | |
Source | PubChem | |
URL | https://pubchem.ncbi.nlm.nih.gov | |
Description | Data deposited in or computed by PubChem | |
Molecular Formula |
C29H38N2O6 | |
Details | Computed by PubChem 2.1 (PubChem release 2021.05.07) | |
Source | PubChem | |
URL | https://pubchem.ncbi.nlm.nih.gov | |
Description | Data deposited in or computed by PubChem | |
Molecular Weight |
510.6 g/mol | |
Details | Computed by PubChem 2.1 (PubChem release 2021.05.07) | |
Source | PubChem | |
URL | https://pubchem.ncbi.nlm.nih.gov | |
Description | Data deposited in or computed by PubChem | |
Retrosynthesis Analysis
AI-Powered Synthesis Planning: Our tool employs the Template_relevance Pistachio, Template_relevance Bkms_metabolic, Template_relevance Pistachio_ringbreaker, Template_relevance Reaxys, Template_relevance Reaxys_biocatalysis model, leveraging a vast database of chemical reactions to predict feasible synthetic routes.
One-Step Synthesis Focus: Specifically designed for one-step synthesis, it provides concise and direct routes for your target compounds, streamlining the synthesis process.
Accurate Predictions: Utilizing the extensive PISTACHIO, BKMS_METABOLIC, PISTACHIO_RINGBREAKER, REAXYS, REAXYS_BIOCATALYSIS database, our tool offers high-accuracy predictions, reflecting the latest in chemical research and data.
Strategy Settings
Precursor scoring | Relevance Heuristic |
---|---|
Min. plausibility | 0.01 |
Model | Template_relevance |
Template Set | Pistachio/Bkms_metabolic/Pistachio_ringbreaker/Reaxys/Reaxys_biocatalysis |
Top-N result to add to graph | 6 |
Feasible Synthetic Routes
Disclaimer and Information on In-Vitro Research Products
Please be aware that all articles and product information presented on BenchChem are intended solely for informational purposes. The products available for purchase on BenchChem are specifically designed for in-vitro studies, which are conducted outside of living organisms. In-vitro studies, derived from the Latin term "in glass," involve experiments performed in controlled laboratory settings using cells or tissues. It is important to note that these products are not categorized as medicines or drugs, and they have not received approval from the FDA for the prevention, treatment, or cure of any medical condition, ailment, or disease. We must emphasize that any form of bodily introduction of these products into humans or animals is strictly prohibited by law. It is essential to adhere to these guidelines to ensure compliance with legal and ethical standards in research and experimentation.