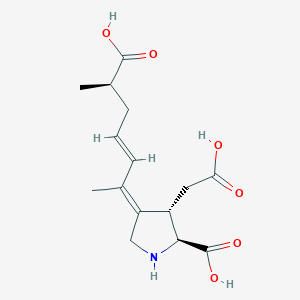
Isodomoic acid G
Overview
Description
Isodomoic acid G (Iso-G) is a neuroexcitatory marine natural product belonging to the kainoid family, which includes domoic acid (DA) and other isodomoic acid isomers. It was first isolated from the red alga Chondria armata and later identified in diatoms such as Pseudo-nitzschia multiseries . Structurally, Iso-G features a 3-carboxymethylproline core with a conjugated hexadienoic acid side chain. Its stereochemistry, particularly the 5'R configuration, was confirmed through total synthesis and comparative circular dichroism (CD) analysis with authentic material . Iso-G exhibits neurotoxicity by agonizing ionotropic glutamate receptors, though its potency is distinct from DA due to differences in side-chain double-bond geometry .
Preparation Methods
Synthetic Routes and Reaction Conditions: The total synthesis of isodomoic acid G involves a series of complex steps. One notable method includes a nickel-catalyzed cyclization that constructs the pyrrolidine ring while establishing the stereochemistry of an exocyclic tetrasubstituted alkene. This process involves the stereoselective assembly of both E- and Z-alkenes of the natural products . Another approach involves a sequential silylcarbocyclization and silicon-based cross-coupling process, which includes key transformations such as a diastereoselective rhodium-catalyzed carbonylative silylcarbocyclization reaction of an L-vinylglycine-derived 1,6-enyne .
Industrial Production Methods: Industrial production of this compound is not well-documented, likely due to the complexity and specificity of the synthetic routes required. Most production methods are confined to laboratory settings and involve intricate organic synthesis techniques.
Chemical Reactions Analysis
Types of Reactions: Isodomoic acid G undergoes various chemical reactions, including:
Oxidation: This reaction can be facilitated by reagents such as potassium permanganate or chromium trioxide.
Reduction: Common reducing agents include lithium aluminum hydride and sodium borohydride.
Substitution: Nucleophilic substitution reactions can occur, particularly at the alkene and pyrrolidine ring positions.
Common Reagents and Conditions:
Oxidation: Potassium permanganate in an acidic medium.
Reduction: Lithium aluminum hydride in dry ether.
Substitution: Sodium methoxide in methanol.
Major Products Formed: The major products formed from these reactions depend on the specific conditions and reagents used. For instance, oxidation may yield carboxylic acids, while reduction can produce alcohols or amines.
Scientific Research Applications
Chemical Synthesis
The synthesis of isodomoic acids, including IA-G, has been a significant area of research. Various synthetic routes have been developed to produce IA-G efficiently:
- Total Synthesis : The first total synthesis of IA-G was accomplished using a silicon-based cross-coupling method. This approach allows for the construction of C-C bonds under mild conditions, making it suitable for late-stage modifications in complex organic syntheses .
- Stereocontrol : The synthesis involves a diastereoselective rhodium-catalyzed carbonylative silylcarbocyclization reaction, which is crucial for achieving the desired stereochemistry in IA-G .
Neurobiological Research
IA-G is structurally related to domoic acid (DA), a potent neurotoxin associated with amnesic shellfish poisoning. Research indicates that IA-G exhibits neuroexcitatory properties similar to DA, which positions it as a valuable model for studying neurodegenerative diseases:
- Neuronal Degeneration : Studies suggest that IA-G induces neuronal degeneration akin to conditions such as Huntington's disease and epilepsy. This makes it a candidate for pharmacological studies aimed at understanding these diseases' pathogenesis .
- Mechanistic Insights : Investigations into the mechanisms by which IA-G affects neuronal cells could provide insights into potential therapeutic targets for neurodegenerative disorders .
The biological activity of IA-G extends beyond neurotoxicity:
- Insecticidal Properties : Some studies have indicated that isodomoic acids, including IA-G, possess insecticidal activity, which may have applications in pest control .
- Chiral Synthons : IA-G can serve as a chiral synthon or building block in the design of peptidomimetics and other bioactive compounds, enhancing its utility in medicinal chemistry .
Analytical Applications
The detection and quantification of IA-G in environmental samples are critical for assessing its ecological impact:
- Analytical Methods : Techniques such as high-performance liquid chromatography (HPLC) and gas chromatography (GC) are employed to analyze IA-G levels in marine environments and shellfish tissues. These methods are essential for monitoring harmful algal blooms and ensuring food safety .
Data Table: Summary of Applications
Case Studies
- Neurotoxicity Assessment : A study demonstrated that IA-G could induce apoptosis in neuronal cell lines, providing a model for understanding the mechanisms underlying neurodegenerative diseases .
- Synthesis Optimization : Researchers optimized the total synthesis route for IA-G to enhance yield and reduce synthesis time, showcasing the compound's potential for large-scale production .
- Environmental Monitoring : A case study on the prevalence of IA-G in coastal waters highlighted its correlation with harmful algal blooms, emphasizing the need for regular monitoring to protect public health .
Mechanism of Action
Isodomoic acid G exerts its effects by acting as an agonist at kainate receptors, which are a subtype of ionotropic glutamate receptors. These receptors are involved in excitatory neurotransmission in the central nervous system. The binding of this compound to these receptors leads to an influx of calcium ions, resulting in neuronal excitation and, at high concentrations, neurotoxicity .
Comparison with Similar Compounds
Structural and Stereochemical Differences
Iso-G is part of a family of isodomoic acids (A–H) and domoic acid derivatives. Key distinctions arise from the position and stereochemistry of exocyclic alkenes and substituents:
Key Observations :
- Alkene Geometry : Iso-G and Iso-H differ in the stereochemistry of their tetrasubstituted alkenes (E vs. Z), controlled during synthesis by altering the timing of methyl/alkenyl group introduction .
- Stereochemical Control : Total syntheses by Montgomery et al. and Denmark et al. highlight divergent strategies:
Data Tables
Table 2: Toxicity Profiles
Compound | LD50 (mg/kg, mice) | Receptor Affinity (KA) | Neuroexcitatory Potency |
---|---|---|---|
Domoic acid | 3.6 | High | ++++ |
This compound | Not reported | Moderate | ++ |
Isodomoic acid A | >10 | Low | + |
Biological Activity
Isodomoic acid G is a member of the kainoid family of compounds, which are primarily derived from marine algae and exhibit significant biological activity, particularly as neuroexcitatory agents. This article delves into the biological properties, synthesis, and mechanisms of action of this compound, supported by data tables and relevant case studies.
Overview of this compound
This compound is structurally related to domoic acid, a potent neurotoxin known to cause amnesic shellfish poisoning (ASP). These compounds act as analogs of glutamic acid, a crucial neurotransmitter in the mammalian central nervous system, and interact with ionotropic glutamate receptors (iGluRs), leading to neuronal excitotoxicity and cell death .
Biological Activity
Mechanism of Action:
this compound mimics glutamate and binds to iGluRs, particularly the AMPA receptor subtype. This binding results in increased calcium influx into neurons, promoting depolarization and potentially leading to excitotoxicity. The compound's ability to induce neuronal death has been documented in various studies, highlighting its relevance in neuropharmacology .
Toxicological Profile:
The toxic effects of this compound are primarily observed in marine organisms and can also extend to mammals through the consumption of contaminated shellfish. The compound exhibits strong insecticidal properties against species like the American cockroach (Periplaneta americana), suggesting a broader ecological impact beyond human health concerns .
Synthesis of this compound
The total synthesis of this compound has been achieved through various synthetic routes involving complex organic reactions. Notably, a silicon-based cross-coupling method has been employed to efficiently construct its molecular framework. The synthesis typically involves:
- Core Fragment Construction: Utilizing a diastereoselective rhodium-catalyzed carbonylative reaction.
- Side Chain Assembly: Achieved via silylcarbocyclization followed by desilylative iodination.
- Final Coupling: A silicon-based alkenyl-alkenyl cross-coupling reaction completes the synthesis .
Case Study 1: Neurotoxicity Assessment
A study investigated the neurotoxic effects of this compound on rat cortical neurons. Results indicated that exposure led to significant neuronal loss and increased markers of oxidative stress, supporting its role as an excitotoxin. The research emphasized the need for monitoring marine biotoxins in seafood to prevent ASP incidents .
Case Study 2: Ecological Impact
Research on algal blooms revealed that species producing isodomoic acids contribute to ecosystem imbalances due to their neurotoxic effects on marine life. This study highlighted the implications for food webs and fisheries, underscoring the importance of understanding these compounds' biological activities in environmental contexts .
Data Tables
Property | This compound |
---|---|
Molecular Formula | C₁₃H₁₅NO₄ |
Molecular Weight | 253.26 g/mol |
Toxicity | Neurotoxic (causes depolarization) |
Source | Marine algae (e.g., Chondria armata) |
Biological Effect | Observation |
---|---|
Neuronal Excitotoxicity | Induces cell death in neurons |
Insecticidal Activity | Effective against Periplaneta americana |
Glutamate Mimicry | Binds to iGluRs |
Q & A
Q. Basic: What experimental strategies are employed in the total synthesis of isodomoic acid G?
Answer: The total synthesis of this compound involves multi-step organic reactions, including stereocontrolled cyclization, oxidation, and functional group transformations. Key steps include:
- Palladium-catalyzed cross-coupling to assemble the pyrrolidine core .
- Dess-Martin periodinane oxidation followed by NaClO₂-mediated oxidation to install carboxylic acid groups .
- Chiral resolution using ion-exchange chromatography to isolate the 5′(R)-isomer, confirmed via CD spectroscopy .
Critical intermediates (e.g., alkenyl iodides and conjugated enynes) are synthesized with >70% yield and stereochemical fidelity .
Q. Basic: How is the structure of this compound confirmed post-synthesis?
Answer: Structural validation combines 1D/2D NMR (e.g., NOESY for stereochemistry) and circular dichroism (CD) spectroscopy. For example:
- 1H NMR distinguishes 5′(R) and 5′(S) isomers via subtle shifts in methyl and pyrrolidine proton signals .
- CD spectra match synthetic 5′(R)-isodomoic acid G with the natural product, confirming absolute configuration .
- High-resolution mass spectrometry (HRMS) validates molecular formula .
Q. Basic: What biosynthetic precursors contribute to this compound in diatoms?
Answer: this compound biosynthesis in Pseudo-nitzschia spp. involves:
- N-prenylation of L-glutamic acid with geranyl pyrophosphate (GPP) .
- Two oxygenase-mediated transformations to form the pyrrolidine ring and conjugated diene .
Gene clusters encoding these enzymes (e.g., cytochrome P450s) are upregulated during toxin production .
Q. Basic: What analytical methods quantify this compound in marine samples?
Answer: Liquid chromatography-tandem mass spectrometry (LC-MS/MS) is the gold standard:
- Dispersive solid-phase extraction pre-concentrates toxins from shellfish .
- Monolithic columns enable high-throughput separation of isomers (e.g., isodomoic acids D, E, F) .
- CID fragmentation patterns differentiate this compound from domoic acid using reference standards .
Q. Advanced: How can stereochemical inversions during synthesis be minimized?
Answer: Strategies include:
- Protecting group selection : TIPS groups prevent undesired carbonyl interactions during iodination, retaining double bond geometry .
- Low-temperature reactions : Pd₂(dba)₃ catalysis at −78°C suppresses epimerization .
- Sodium amalgam optimization : Prevents diene reduction during deprotection .
Q. Advanced: How to resolve discrepancies in NMR data between synthetic and natural this compound?
Answer: Discrepancies often arise from:
- Impurity profiling : Use preparative HPLC to isolate trace isomers (e.g., 5′(S)-isomer) .
- Dynamic NMR studies : Assess conformational exchange in pyrrolidine rings at variable temperatures .
- Mixed-sample analysis : Compare synthetic and natural product spectra to identify non-identical signals .
Q. Advanced: How do salinity gradients affect this compound production in diatoms?
Answer: Salinity stress experiments reveal:
- Optimal production at 30–40 psu in Pseudo-nitzschia seriata, linked to osmoregulatory gene activation .
- LC-MS quantification shows 2.5× higher cellular toxin levels at 40 psu vs. 20 psu .
- Nutrient co-limitation (e.g., silicate) modulates toxin yields under hypersaline conditions .
Q. Advanced: What distinguishes the neurotoxic potency of this compound from its isomers?
Answer: In vivo murine models demonstrate:
- Seizure induction : this compound requires 1.8× higher doses than domoic acid (ED₅₀ = 2.1 mg/kg vs. 1.2 mg/kg) .
- Receptor affinity : Competitive binding assays show 10× lower affinity for kainate receptors vs. domoic acid .
- Metabolite profiling : Glutathione conjugation rates differ among isomers, altering detoxification pathways .
Q. Advanced: What enzymatic mechanisms enable C–H activation in this compound biosynthesis?
Answer: Non-heme iron oxygenases catalyze:
- Radical-mediated cyclization : Install the pyrrolidine ring via tyrosine-valine crosslinking .
- Desaturation : Create conjugated dienes using Fe(IV)-oxo intermediates .
In vitro reconstitution with isotopically labeled substrates confirms these steps .
Q. Advanced: What challenges arise in distinguishing this compound from epi-DA in environmental samples?
Answer: Co-elution in HPLC and similar CID spectra require:
- Ion mobility spectrometry (IMS) : Resolve isomers via collision cross-section differences .
- NMR-guided isolation : Compare ¹³C chemical shifts at C5′ (δ = 72.1 ppm for this compound vs. 68.9 ppm for epi-DA) .
- Species-specific profiling : Correlate toxin ratios with diatom strain genetics (e.g., P. plurisecta vs. N. naviculoides) .
Properties
IUPAC Name |
(2S,3S,4E)-4-[(E,6R)-6-carboxyhept-3-en-2-ylidene]-3-(carboxymethyl)pyrrolidine-2-carboxylic acid | |
---|---|---|
Details | Computed by LexiChem 2.6.6 (PubChem release 2019.06.18) | |
Source | PubChem | |
URL | https://pubchem.ncbi.nlm.nih.gov | |
Description | Data deposited in or computed by PubChem | |
InChI |
InChI=1S/C15H21NO6/c1-8(4-3-5-9(2)14(19)20)11-7-16-13(15(21)22)10(11)6-12(17)18/h3-4,9-10,13,16H,5-7H2,1-2H3,(H,17,18)(H,19,20)(H,21,22)/b4-3+,11-8-/t9-,10+,13+/m1/s1 | |
Details | Computed by InChI 1.0.5 (PubChem release 2019.06.18) | |
Source | PubChem | |
URL | https://pubchem.ncbi.nlm.nih.gov | |
Description | Data deposited in or computed by PubChem | |
InChI Key |
MKCCCBSBSRCARB-NMWGDLGCSA-N | |
Details | Computed by InChI 1.0.5 (PubChem release 2019.06.18) | |
Source | PubChem | |
URL | https://pubchem.ncbi.nlm.nih.gov | |
Description | Data deposited in or computed by PubChem | |
Canonical SMILES |
CC(CC=CC(=C1CNC(C1CC(=O)O)C(=O)O)C)C(=O)O | |
Details | Computed by OEChem 2.1.5 (PubChem release 2019.06.18) | |
Source | PubChem | |
URL | https://pubchem.ncbi.nlm.nih.gov | |
Description | Data deposited in or computed by PubChem | |
Isomeric SMILES |
C[C@H](C/C=C/C(=C\1/CN[C@@H]([C@H]1CC(=O)O)C(=O)O)/C)C(=O)O | |
Details | Computed by OEChem 2.1.5 (PubChem release 2019.06.18) | |
Source | PubChem | |
URL | https://pubchem.ncbi.nlm.nih.gov | |
Description | Data deposited in or computed by PubChem | |
Molecular Formula |
C15H21NO6 | |
Details | Computed by PubChem 2.1 (PubChem release 2019.06.18) | |
Source | PubChem | |
URL | https://pubchem.ncbi.nlm.nih.gov | |
Description | Data deposited in or computed by PubChem | |
Molecular Weight |
311.33 g/mol | |
Details | Computed by PubChem 2.1 (PubChem release 2021.05.07) | |
Source | PubChem | |
URL | https://pubchem.ncbi.nlm.nih.gov | |
Description | Data deposited in or computed by PubChem | |
Retrosynthesis Analysis
AI-Powered Synthesis Planning: Our tool employs the Template_relevance Pistachio, Template_relevance Bkms_metabolic, Template_relevance Pistachio_ringbreaker, Template_relevance Reaxys, Template_relevance Reaxys_biocatalysis model, leveraging a vast database of chemical reactions to predict feasible synthetic routes.
One-Step Synthesis Focus: Specifically designed for one-step synthesis, it provides concise and direct routes for your target compounds, streamlining the synthesis process.
Accurate Predictions: Utilizing the extensive PISTACHIO, BKMS_METABOLIC, PISTACHIO_RINGBREAKER, REAXYS, REAXYS_BIOCATALYSIS database, our tool offers high-accuracy predictions, reflecting the latest in chemical research and data.
Strategy Settings
Precursor scoring | Relevance Heuristic |
---|---|
Min. plausibility | 0.01 |
Model | Template_relevance |
Template Set | Pistachio/Bkms_metabolic/Pistachio_ringbreaker/Reaxys/Reaxys_biocatalysis |
Top-N result to add to graph | 6 |
Feasible Synthetic Routes
Disclaimer and Information on In-Vitro Research Products
Please be aware that all articles and product information presented on BenchChem are intended solely for informational purposes. The products available for purchase on BenchChem are specifically designed for in-vitro studies, which are conducted outside of living organisms. In-vitro studies, derived from the Latin term "in glass," involve experiments performed in controlled laboratory settings using cells or tissues. It is important to note that these products are not categorized as medicines or drugs, and they have not received approval from the FDA for the prevention, treatment, or cure of any medical condition, ailment, or disease. We must emphasize that any form of bodily introduction of these products into humans or animals is strictly prohibited by law. It is essential to adhere to these guidelines to ensure compliance with legal and ethical standards in research and experimentation.