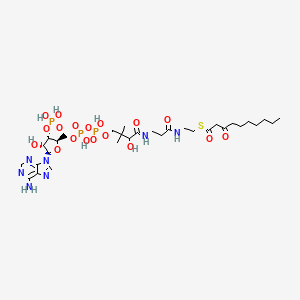
3-ketodecanoyl-CoA
Overview
Description
3-Ketodecanoyl-CoA is a thioester formed between 3-ketodecanoic acid and coenzyme A. It serves as a key intermediate in the fatty acid β-oxidation pathway, particularly within peroxisomes. This metabolic pathway is crucial for breaking down fatty acids to generate energy.
Mechanism of Action
Target of Action
The primary targets of 3-ketodecanoyl-CoA are enzymes involved in the β-oxidation cycle, specifically the enoyl-CoA hydratase and 3-hydroxyacyl-CoA dehydrogenase . These enzymes are associated with a multifunctional polypeptide and are located on the large subunit of the fatty acid oxidation complex . They play a crucial role in the breakdown of fatty acids, a process that provides energy for cellular functions.
Mode of Action
This compound interacts with its targets through a series of biochemical reactions. The compound is involved in the Claisen condensation reaction between two acyl-CoAs, catalyzed by the 3-ketoacyl-CoA thiolase (KAT), a member of the thiolase family . This reaction results in the elongation of the carbon chain .
Biochemical Pathways
This compound is a key intermediate in the β-oxidation pathway . This metabolic pathway involves the breakdown of fatty acids into acetyl-CoA, which can then enter the citric acid cycle to produce energy. The conversion of 2-decenoyl-CoA to this compound is catalyzed by the sequential action of the hydratase and dehydrogenase of the complex .
Result of Action
The action of this compound results in the production of energy within cells. By participating in the β-oxidation pathway, it contributes to the breakdown of fatty acids and the generation of acetyl-CoA . This molecule can then enter the citric acid cycle, leading to the production of ATP, the primary energy currency of the cell.
Action Environment
The action of this compound is influenced by various environmental factors within the cell. For instance, the availability of other substrates and cofactors, such as NAD+ and CoA, can affect the efficiency of the β-oxidation pathway . Additionally, the overall metabolic state of the cell, including the balance between energy production and consumption, can also influence the action of this compound.
Biochemical Analysis
Biochemical Properties
3-Ketodecanoyl-CoA is involved in the beta-oxidation pathway, where it serves as a substrate for medium-chain acyl-CoA dehydrogenase. This enzyme catalyzes the dehydrogenation of this compound, creating a double bond between the alpha and beta carbons . The hydrogen acceptor in this reaction is flavin adenine dinucleotide (FAD), which is reduced to FADH2. Additionally, this compound interacts with other enzymes such as 3-ketoacyl-CoA thiolase, which catalyzes the Claisen condensation reaction between two acyl-CoAs, thereby achieving carbon chain elongation .
Cellular Effects
This compound influences various cellular processes, including cell signaling pathways, gene expression, and cellular metabolism. It is involved in the beta-oxidation cycle, which is essential for energy production in cells. The compound’s role in fatty acid metabolism affects the overall metabolic flux and energy balance within the cell . Additionally, this compound has been shown to interact with enzymes involved in the synthesis of very long-chain fatty acids, impacting the composition of cell membranes and signaling molecules .
Molecular Mechanism
At the molecular level, this compound exerts its effects through binding interactions with various enzymes and biomolecules. It acts as a substrate for medium-chain acyl-CoA dehydrogenase, facilitating the dehydrogenation process in the beta-oxidation pathway . The compound also interacts with 3-ketoacyl-CoA thiolase, which catalyzes the Claisen condensation reaction, leading to carbon chain elongation . These interactions are crucial for maintaining cellular energy homeostasis and metabolic balance.
Temporal Effects in Laboratory Settings
In laboratory settings, the stability and degradation of this compound have been studied to understand its long-term effects on cellular function. The compound is relatively stable under controlled conditions, but its degradation can lead to changes in cellular metabolism over time . In vitro and in vivo studies have shown that prolonged exposure to this compound can affect the beta-oxidation pathway and energy production in cells .
Dosage Effects in Animal Models
The effects of this compound vary with different dosages in animal models. At low doses, the compound supports normal metabolic functions and energy production. At high doses, it can lead to toxic effects and disrupt cellular metabolism . Studies have shown that excessive levels of this compound can cause oxidative stress and damage to cellular components, highlighting the importance of maintaining appropriate dosage levels .
Metabolic Pathways
This compound is a key intermediate in the beta-oxidation pathway, where it undergoes dehydrogenation by medium-chain acyl-CoA dehydrogenase . This process is essential for the breakdown of fatty acids and the production of energy in the form of adenosine triphosphate (ATP). The compound also participates in the synthesis of very long-chain fatty acids, which are important for cell membrane integrity and signaling .
Transport and Distribution
Within cells, this compound is transported and distributed by specific transporters and binding proteins. These molecules facilitate the movement of the compound to various cellular compartments where it is needed for metabolic processes . The localization and accumulation of this compound are regulated by its interactions with enzymes and transporters involved in fatty acid metabolism .
Subcellular Localization
This compound is primarily localized in the mitochondria, where it participates in the beta-oxidation pathway . The compound’s activity and function are influenced by its subcellular localization, as it interacts with mitochondrial enzymes to facilitate energy production. Additionally, this compound may be found in the cytosol and peroxisomes, where it plays a role in fatty acid synthesis and degradation .
Preparation Methods
Synthetic Routes and Reaction Conditions: 3-Ketodecanoyl-CoA can be synthesized through the sequential action of enoyl-CoA hydratase and 3-hydroxyacyl-CoA dehydrogenase. The conversion of 2-decenoyl-CoA to this compound is catalyzed by these enzymes, and the rate of NADH formation is measured to determine the reaction progress .
Chemical Reactions Analysis
Types of Reactions: 3-Ketodecanoyl-CoA undergoes various reactions, including:
Oxidation: It can be oxidized to form other intermediates in the β-oxidation pathway.
Reduction: It can be reduced to form 3-hydroxydecanoyl-CoA.
Substitution: It can participate in substitution reactions with other acyl-CoA derivatives.
Common Reagents and Conditions:
Oxidation: Requires NAD+ as a cofactor.
Reduction: Requires NADH as a cofactor.
Substitution: Typically involves other acyl-CoA derivatives and specific enzymes.
Major Products:
Scientific Research Applications
3-Ketodecanoyl-CoA has several scientific research applications:
Chemistry: It is used to study the mechanisms of fatty acid β-oxidation and enzyme kinetics.
Biology: It serves as a model compound to understand metabolic pathways in cells.
Medicine: It is used in research related to metabolic disorders and energy production.
Industry: It is utilized in the production of biofuels and other value-added chemicals through metabolic engineering .
Comparison with Similar Compounds
3-Hydroxydecanoyl-CoA: A reduced form of 3-ketodecanoyl-CoA.
3-Ketohexanoyl-CoA: A shorter-chain analog.
3-Ketooctanoyl-CoA: Another shorter-chain analog.
Uniqueness: this compound is unique due to its specific chain length and its role as an intermediate in the β-oxidation of fatty acids. Its structure allows it to participate in specific enzymatic reactions that are crucial for energy production in cells .
Properties
IUPAC Name |
S-[2-[3-[[4-[[[(2R,3S,4R,5R)-5-(6-aminopurin-9-yl)-4-hydroxy-3-phosphonooxyoxolan-2-yl]methoxy-hydroxyphosphoryl]oxy-hydroxyphosphoryl]oxy-2-hydroxy-3,3-dimethylbutanoyl]amino]propanoylamino]ethyl] 3-oxodecanethioate | |
---|---|---|
Details | Computed by Lexichem TK 2.7.0 (PubChem release 2021.05.07) | |
Source | PubChem | |
URL | https://pubchem.ncbi.nlm.nih.gov | |
Description | Data deposited in or computed by PubChem | |
InChI |
InChI=1S/C31H52N7O18P3S/c1-4-5-6-7-8-9-19(39)14-22(41)60-13-12-33-21(40)10-11-34-29(44)26(43)31(2,3)16-53-59(50,51)56-58(48,49)52-15-20-25(55-57(45,46)47)24(42)30(54-20)38-18-37-23-27(32)35-17-36-28(23)38/h17-18,20,24-26,30,42-43H,4-16H2,1-3H3,(H,33,40)(H,34,44)(H,48,49)(H,50,51)(H2,32,35,36)(H2,45,46,47)/t20-,24-,25-,26?,30-/m1/s1 | |
Details | Computed by InChI 1.0.6 (PubChem release 2021.05.07) | |
Source | PubChem | |
URL | https://pubchem.ncbi.nlm.nih.gov | |
Description | Data deposited in or computed by PubChem | |
InChI Key |
AZCVXMAPLHSIKY-BOJFXZHGSA-N | |
Details | Computed by InChI 1.0.6 (PubChem release 2021.05.07) | |
Source | PubChem | |
URL | https://pubchem.ncbi.nlm.nih.gov | |
Description | Data deposited in or computed by PubChem | |
Canonical SMILES |
CCCCCCCC(=O)CC(=O)SCCNC(=O)CCNC(=O)C(C(C)(C)COP(=O)(O)OP(=O)(O)OCC1C(C(C(O1)N2C=NC3=C(N=CN=C32)N)O)OP(=O)(O)O)O | |
Details | Computed by OEChem 2.3.0 (PubChem release 2021.05.07) | |
Source | PubChem | |
URL | https://pubchem.ncbi.nlm.nih.gov | |
Description | Data deposited in or computed by PubChem | |
Isomeric SMILES |
CCCCCCCC(=O)CC(=O)SCCNC(=O)CCNC(=O)C(C(C)(C)COP(=O)(O)OP(=O)(O)OC[C@@H]1[C@H]([C@H]([C@@H](O1)N2C=NC3=C(N=CN=C32)N)O)OP(=O)(O)O)O | |
Details | Computed by OEChem 2.3.0 (PubChem release 2021.05.07) | |
Source | PubChem | |
URL | https://pubchem.ncbi.nlm.nih.gov | |
Description | Data deposited in or computed by PubChem | |
Molecular Formula |
C31H52N7O18P3S | |
Details | Computed by PubChem 2.1 (PubChem release 2021.05.07) | |
Source | PubChem | |
URL | https://pubchem.ncbi.nlm.nih.gov | |
Description | Data deposited in or computed by PubChem | |
DSSTOX Substance ID |
DTXSID80331506 | |
Record name | 3-ketodecanoyl-CoA | |
Source | EPA DSSTox | |
URL | https://comptox.epa.gov/dashboard/DTXSID80331506 | |
Description | DSSTox provides a high quality public chemistry resource for supporting improved predictive toxicology. | |
Molecular Weight |
935.8 g/mol | |
Details | Computed by PubChem 2.1 (PubChem release 2021.05.07) | |
Source | PubChem | |
URL | https://pubchem.ncbi.nlm.nih.gov | |
Description | Data deposited in or computed by PubChem | |
Physical Description |
Solid | |
Record name | 3-Oxodecanoyl-CoA | |
Source | Human Metabolome Database (HMDB) | |
URL | http://www.hmdb.ca/metabolites/HMDB0003939 | |
Description | The Human Metabolome Database (HMDB) is a freely available electronic database containing detailed information about small molecule metabolites found in the human body. | |
Explanation | HMDB is offered to the public as a freely available resource. Use and re-distribution of the data, in whole or in part, for commercial purposes requires explicit permission of the authors and explicit acknowledgment of the source material (HMDB) and the original publication (see the HMDB citing page). We ask that users who download significant portions of the database cite the HMDB paper in any resulting publications. | |
CAS No. |
50411-91-1 | |
Record name | 3-ketodecanoyl-CoA | |
Source | EPA DSSTox | |
URL | https://comptox.epa.gov/dashboard/DTXSID80331506 | |
Description | DSSTox provides a high quality public chemistry resource for supporting improved predictive toxicology. | |
Record name | 3-Oxodecanoyl-CoA | |
Source | Human Metabolome Database (HMDB) | |
URL | http://www.hmdb.ca/metabolites/HMDB0003939 | |
Description | The Human Metabolome Database (HMDB) is a freely available electronic database containing detailed information about small molecule metabolites found in the human body. | |
Explanation | HMDB is offered to the public as a freely available resource. Use and re-distribution of the data, in whole or in part, for commercial purposes requires explicit permission of the authors and explicit acknowledgment of the source material (HMDB) and the original publication (see the HMDB citing page). We ask that users who download significant portions of the database cite the HMDB paper in any resulting publications. | |
Retrosynthesis Analysis
AI-Powered Synthesis Planning: Our tool employs the Template_relevance Pistachio, Template_relevance Bkms_metabolic, Template_relevance Pistachio_ringbreaker, Template_relevance Reaxys, Template_relevance Reaxys_biocatalysis model, leveraging a vast database of chemical reactions to predict feasible synthetic routes.
One-Step Synthesis Focus: Specifically designed for one-step synthesis, it provides concise and direct routes for your target compounds, streamlining the synthesis process.
Accurate Predictions: Utilizing the extensive PISTACHIO, BKMS_METABOLIC, PISTACHIO_RINGBREAKER, REAXYS, REAXYS_BIOCATALYSIS database, our tool offers high-accuracy predictions, reflecting the latest in chemical research and data.
Strategy Settings
Precursor scoring | Relevance Heuristic |
---|---|
Min. plausibility | 0.01 |
Model | Template_relevance |
Template Set | Pistachio/Bkms_metabolic/Pistachio_ringbreaker/Reaxys/Reaxys_biocatalysis |
Top-N result to add to graph | 6 |
Feasible Synthetic Routes
Q1: How does 3-ketodecanoyl-CoA interact with rat peroxisomal multifunctional enzyme type 1 (rMFE1)?
A1: this compound binds to the hydroxyacyl-CoA dehydrogenase (HAD) active site of rMFE1. This interaction involves residues within the C, D, and E domains of the enzyme. [1] Crystallographic studies have revealed the specific interactions between this compound and the amino acids in these domains, providing insight into the structural basis of substrate recognition and binding. [1, 3, 4]
Q2: What is the significance of capturing both this compound and NAD+ bound to the HAD active site of rMFE1?
A2: Crystallizing rMFE1 with both this compound and NAD+ provides a snapshot of the enzyme in a catalytically relevant state. This allows researchers to visualize the enzyme-substrate-cofactor complex and understand the interactions that facilitate the dehydrogenase reaction. [1, 3, 4] This information is crucial for elucidating the catalytic mechanism of rMFE1 and its role in fatty acid β-oxidation within peroxisomes.
Q3: Does the binding of this compound to rMFE1 induce any conformational changes?
A3: Yes, structural comparisons of rMFE1 in different states suggest that this compound binding leads to domain movements within the enzyme. Notably, the C domain shifts relative to the D/E domains, and the A domain moves with respect to the HAD portion of the enzyme. [1] These conformational changes are likely essential for substrate binding, product release, and overall catalytic efficiency.
Q4: What is the role of the linker helix in rMFE1 activity?
A4: The linker helix in rMFE1, specifically the N-terminal part interacting with domains A and E, appears to act as a hinge. [1] This hinge-like function facilitates the movement of the A domain relative to the HAD portion of the enzyme. This movement is thought to be crucial for the catalytic cycle, potentially influencing substrate access, product release, or domain rearrangements required for subsequent steps in the β-oxidation pathway.
Disclaimer and Information on In-Vitro Research Products
Please be aware that all articles and product information presented on BenchChem are intended solely for informational purposes. The products available for purchase on BenchChem are specifically designed for in-vitro studies, which are conducted outside of living organisms. In-vitro studies, derived from the Latin term "in glass," involve experiments performed in controlled laboratory settings using cells or tissues. It is important to note that these products are not categorized as medicines or drugs, and they have not received approval from the FDA for the prevention, treatment, or cure of any medical condition, ailment, or disease. We must emphasize that any form of bodily introduction of these products into humans or animals is strictly prohibited by law. It is essential to adhere to these guidelines to ensure compliance with legal and ethical standards in research and experimentation.