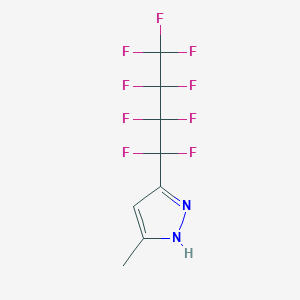
3-(Nonafluoro-1-butyl)-5-(methyl)pyrazole
Overview
Description
3-(Nonafluoro-1-butyl)-5-(methyl)pyrazole is a fluorinated pyrazole derivative. Fluorinated compounds are known for their unique chemical properties, such as high thermal stability, resistance to oxidation, and low reactivity, making them valuable in various industrial and scientific applications. The presence of the nonafluoro-1-butyl group and the methyl group on the pyrazole ring imparts distinct characteristics to this compound.
Preparation Methods
Synthetic Routes and Reaction Conditions
The synthesis of 3-(Nonafluoro-1-butyl)-5-(methyl)pyrazole typically involves the following steps:
Formation of the Pyrazole Ring: The pyrazole ring can be synthesized through the reaction of hydrazine with a 1,3-dicarbonyl compound, such as acetylacetone, under acidic or basic conditions.
Introduction of the Nonafluoro-1-butyl Group: The nonafluoro-1-butyl group can be introduced through a nucleophilic substitution reaction using a suitable fluorinated alkyl halide, such as nonafluoro-1-butyl iodide, in the presence of a base like potassium carbonate.
Methylation: The methyl group can be introduced via a methylation reaction using a methylating agent, such as methyl iodide, in the presence of a base like sodium hydride.
Industrial Production Methods
Industrial production of this compound may involve optimized versions of the above synthetic routes, with considerations for scalability, cost-effectiveness, and environmental impact. Continuous flow reactors and automated synthesis platforms can be employed to enhance efficiency and yield.
Chemical Reactions Analysis
Types of Reactions
3-(Nonafluoro-1-butyl)-5-(methyl)pyrazole can undergo various chemical reactions, including:
Oxidation: The compound can be oxidized using oxidizing agents like potassium permanganate or hydrogen peroxide, leading to the formation of oxidized derivatives.
Reduction: Reduction reactions can be carried out using reducing agents such as lithium aluminum hydride or sodium borohydride, resulting in reduced forms of the compound.
Substitution: The fluorinated alkyl group can participate in nucleophilic substitution reactions with nucleophiles like amines or thiols, leading to the formation of substituted derivatives.
Common Reagents and Conditions
Oxidation: Potassium permanganate in acidic or neutral conditions.
Reduction: Lithium aluminum hydride in anhydrous ether.
Substitution: Nucleophiles like amines in the presence of a base such as triethylamine.
Major Products
Oxidation: Oxidized derivatives with additional oxygen-containing functional groups.
Reduction: Reduced derivatives with fewer double bonds or oxygen atoms.
Substitution: Substituted derivatives with new functional groups replacing the fluorinated alkyl group.
Scientific Research Applications
3-(Nonafluoro-1-butyl)-5-(methyl)pyrazole has several scientific research applications, including:
Chemistry: Used as a building block for the synthesis of more complex fluorinated compounds and materials.
Biology: Investigated for its potential biological activity, including antimicrobial and anticancer properties.
Medicine: Explored as a potential drug candidate due to its unique chemical properties and biological activity.
Industry: Utilized in the development of advanced materials, such as fluorinated polymers and coatings, due to its high thermal stability and resistance to oxidation.
Mechanism of Action
The mechanism of action of 3-(Nonafluoro-1-butyl)-5-(methyl)pyrazole depends on its specific application. In biological systems, it may interact with molecular targets such as enzymes or receptors, modulating their activity and leading to various physiological effects. The fluorinated alkyl group can enhance the compound’s lipophilicity, facilitating its interaction with lipid membranes and intracellular targets.
Comparison with Similar Compounds
Similar Compounds
3-(Nonafluoro-1-butyl)pyrazole: Lacks the methyl group, resulting in different chemical and biological properties.
5-(Methyl)pyrazole: Lacks the nonafluoro-1-butyl group, leading to reduced thermal stability and resistance to oxidation.
3-(Nonafluoro-1-butyl)-5-(ethyl)pyrazole: Contains an ethyl group instead of a methyl group, affecting its chemical reactivity and biological activity.
Uniqueness
3-(Nonafluoro-1-butyl)-5-(methyl)pyrazole is unique due to the presence of both the nonafluoro-1-butyl group and the methyl group on the pyrazole ring. This combination imparts distinct chemical properties, such as high thermal stability, resistance to oxidation, and unique biological activity, making it valuable for various scientific and industrial applications.
Properties
IUPAC Name |
5-methyl-3-(1,1,2,2,3,3,4,4,4-nonafluorobutyl)-1H-pyrazole | |
---|---|---|
Details | Computed by LexiChem 2.6.6 (PubChem release 2019.06.18) | |
Source | PubChem | |
URL | https://pubchem.ncbi.nlm.nih.gov | |
Description | Data deposited in or computed by PubChem | |
InChI |
InChI=1S/C8H5F9N2/c1-3-2-4(19-18-3)5(9,10)6(11,12)7(13,14)8(15,16)17/h2H,1H3,(H,18,19) | |
Details | Computed by InChI 1.0.5 (PubChem release 2019.06.18) | |
Source | PubChem | |
URL | https://pubchem.ncbi.nlm.nih.gov | |
Description | Data deposited in or computed by PubChem | |
InChI Key |
UEWLDKAYGDLRGH-UHFFFAOYSA-N | |
Details | Computed by InChI 1.0.5 (PubChem release 2019.06.18) | |
Source | PubChem | |
URL | https://pubchem.ncbi.nlm.nih.gov | |
Description | Data deposited in or computed by PubChem | |
Canonical SMILES |
CC1=CC(=NN1)C(C(C(C(F)(F)F)(F)F)(F)F)(F)F | |
Details | Computed by OEChem 2.1.5 (PubChem release 2019.06.18) | |
Source | PubChem | |
URL | https://pubchem.ncbi.nlm.nih.gov | |
Description | Data deposited in or computed by PubChem | |
Molecular Formula |
C8H5F9N2 | |
Details | Computed by PubChem 2.1 (PubChem release 2019.06.18) | |
Source | PubChem | |
URL | https://pubchem.ncbi.nlm.nih.gov | |
Description | Data deposited in or computed by PubChem | |
DSSTOX Substance ID |
DTXSID20379705 | |
Record name | 3-(Nonafluoro-1-butyl)-5-(methyl)pyrazole | |
Source | EPA DSSTox | |
URL | https://comptox.epa.gov/dashboard/DTXSID20379705 | |
Description | DSSTox provides a high quality public chemistry resource for supporting improved predictive toxicology. | |
Molecular Weight |
300.12 g/mol | |
Details | Computed by PubChem 2.1 (PubChem release 2021.05.07) | |
Source | PubChem | |
URL | https://pubchem.ncbi.nlm.nih.gov | |
Description | Data deposited in or computed by PubChem | |
CAS No. |
247220-81-1 | |
Record name | 3-(Nonafluoro-1-butyl)-5-(methyl)pyrazole | |
Source | EPA DSSTox | |
URL | https://comptox.epa.gov/dashboard/DTXSID20379705 | |
Description | DSSTox provides a high quality public chemistry resource for supporting improved predictive toxicology. | |
Retrosynthesis Analysis
AI-Powered Synthesis Planning: Our tool employs the Template_relevance Pistachio, Template_relevance Bkms_metabolic, Template_relevance Pistachio_ringbreaker, Template_relevance Reaxys, Template_relevance Reaxys_biocatalysis model, leveraging a vast database of chemical reactions to predict feasible synthetic routes.
One-Step Synthesis Focus: Specifically designed for one-step synthesis, it provides concise and direct routes for your target compounds, streamlining the synthesis process.
Accurate Predictions: Utilizing the extensive PISTACHIO, BKMS_METABOLIC, PISTACHIO_RINGBREAKER, REAXYS, REAXYS_BIOCATALYSIS database, our tool offers high-accuracy predictions, reflecting the latest in chemical research and data.
Strategy Settings
Precursor scoring | Relevance Heuristic |
---|---|
Min. plausibility | 0.01 |
Model | Template_relevance |
Template Set | Pistachio/Bkms_metabolic/Pistachio_ringbreaker/Reaxys/Reaxys_biocatalysis |
Top-N result to add to graph | 6 |
Feasible Synthetic Routes
Disclaimer and Information on In-Vitro Research Products
Please be aware that all articles and product information presented on BenchChem are intended solely for informational purposes. The products available for purchase on BenchChem are specifically designed for in-vitro studies, which are conducted outside of living organisms. In-vitro studies, derived from the Latin term "in glass," involve experiments performed in controlled laboratory settings using cells or tissues. It is important to note that these products are not categorized as medicines or drugs, and they have not received approval from the FDA for the prevention, treatment, or cure of any medical condition, ailment, or disease. We must emphasize that any form of bodily introduction of these products into humans or animals is strictly prohibited by law. It is essential to adhere to these guidelines to ensure compliance with legal and ethical standards in research and experimentation.