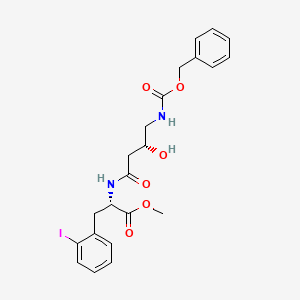
alpha-Helical-corticotropin-releasing factor (9-41)
Overview
Description
α-Helical corticotropin-releasing factor (9-41) (αhCRF(9-41)) is a 30-amino acid peptide antagonist derived from the C-terminal fragment of α-helical CRF, a synthetic analog of corticotropin-releasing factor (CRF). CRF, a 41-residue neuropeptide, regulates the hypothalamic-pituitary-adrenal (HPA) axis and modulates stress responses, anxiety, and appetite . The design of αhCRF(9-41) aimed to optimize α-helicity by substituting residues from CRF family members (e.g., sauvagine, urotensin I) with high α-helix-forming propensity, enhancing receptor binding and stability . In vitro studies demonstrated that αhCRF(9-41) acts primarily as a competitive antagonist at both CRF1 and CRF2 receptors, though weak partial agonism at CRF1 receptors has been observed . In vivo, it inhibits CRF-mediated effects such as ACTH release, tachycardia, and stress-induced behaviors, but its efficacy varies depending on administration route and receptor subtype distribution .
Preparation Methods
Synthetic Routes and Reaction Conditions
The synthesis of alpha-Helical-corticotropin-releasing factor (9-41) involves solid-phase peptide synthesis (SPPS). This method allows for the sequential addition of amino acids to a growing peptide chain anchored to a solid resin. The process typically involves the following steps:
Resin Loading: The first amino acid is attached to the resin.
Deprotection: The protecting group on the amino acid is removed.
Coupling: The next amino acid is activated and coupled to the growing peptide chain.
Repetition: Steps 2 and 3 are repeated until the desired peptide sequence is obtained.
Cleavage: The peptide is cleaved from the resin and purified.
Industrial Production Methods
Industrial production of alpha-Helical-corticotropin-releasing factor (9-41) follows similar principles as laboratory synthesis but on a larger scale. Automated peptide synthesizers are often used to increase efficiency and consistency. The purification process typically involves high-performance liquid chromatography (HPLC) to ensure high purity levels .
Chemical Reactions Analysis
Types of Reactions
Alpha-Helical-corticotropin-releasing factor (9-41) primarily undergoes peptide bond formation and cleavage reactions during its synthesis and degradation. It does not typically participate in oxidation, reduction, or substitution reactions under physiological conditions.
Common Reagents and Conditions
Coupling Reagents: N,N’-Diisopropylcarbodiimide (DIC), O-Benzotriazole-N,N,N’,N’-tetramethyl-uronium-hexafluoro-phosphate (HBTU)
Deprotection Reagents: Trifluoroacetic acid (TFA)
Cleavage Reagents: TFA, water, and scavengers such as triisopropylsilane (TIS).
Major Products
The primary product of the synthesis is the alpha-Helical-corticotropin-releasing factor (9-41) peptide itself. During degradation, the peptide may be broken down into smaller fragments by proteolytic enzymes.
Scientific Research Applications
Key Mechanisms:
- CRF Receptor Antagonism: It binds to CRF receptors, inhibiting their activation and thereby reducing the downstream effects on ACTH secretion and cortisol release.
- Impact on Anxiety: Studies have shown that alpha-helical CRF (9-41) can reverse anxiety-like behaviors induced by various stimuli, including nicotine and stressors.
Research Applications
The applications of alpha-helical CRF (9-41) in scientific research are diverse, with significant findings in behavioral neuroscience, pharmacology, and potential therapeutic uses.
Behavioral Neuroscience
Research has demonstrated that alpha-helical CRF (9-41) can significantly influence motor learning and anxiety responses in animal models.
Case Study: Motor Learning in Rats
- In a study involving rats injected with CRF into the cerebellum, those treated with alpha-helical CRF (9-41) exhibited impaired motor learning compared to controls. This suggests that while CRF enhances performance initially, its antagonism via alpha-helical CRF (9-41) alters this effect negatively .
Treatment Group | Walking Duration (s) | Statistical Significance |
---|---|---|
PBS Control | 20.0 ± 2.8 | - |
CRF Treatment | 56.1 ± 7.2 | p < 0.0001 |
α-h CRF Treatment | 16.1 ± 2.5 | p < 0.0471 |
Pharmacological Studies
Alpha-helical CRF (9-41) has been investigated for its role in reversing anxiety-like effects caused by nicotine and other anxiogenic agents.
Case Study: Nicotine-Induced Anxiety
- A study found that while nicotine induced conditioned anxiety in rats, administration of alpha-helical CRF (9-41) effectively reversed this conditioned response without affecting unconditioned anxiety .
Condition | Anxiety Response | Effect of α-h CRF (9-41) |
---|---|---|
Unconditioned | Yes | No Effect |
Conditioned | Yes | Significant Reversal |
Clinical Implications
The potential therapeutic applications of alpha-helical CRF (9-41) are being explored for conditions such as:
Mechanism of Action
Alpha-Helical-corticotropin-releasing factor (9-41) exerts its effects by binding to corticotropin-releasing factor receptors, thereby blocking the action of endogenous corticotropin-releasing factor. This inhibition prevents the activation of the HPA axis, reducing the release of adrenocorticotropic hormone (ACTH) and subsequent cortisol production . The molecular targets include corticotropin-releasing factor receptor 1 (CRF1) and corticotropin-releasing factor receptor 2 (CRF2) .
Comparison with Similar Compounds
Comparison with Similar CRF-Related Compounds
Structural and Functional Analogues
2.1.1. [DPhe<sup>12</sup>,Nle<sup>21,38</sup>]-hCRF(12–41)
- Structure: A truncated human CRF analog with substitutions (D-Phe<sup>12</sup>, norleucine<sup>21,38</sup>) to enhance stability and receptor affinity.
- Receptor Selectivity : Preferentially binds CRF1 receptors but lacks subtype specificity in functional assays .
2.1.2. Astressin2B
- Structure : Cyclic peptide antagonist with modifications to improve CRF2 receptor selectivity.
- Activity : Potent antagonist at CRF2 receptors (IC50 ~1 nM) but exhibits weak partial agonism at CRF2 in cAMP assays .
2.1.3. D-PheCRF(12-41)
- Structure : Linear peptide antagonist with D-Phe substitution at position 12.
- Activity: Blocks CRF-induced ACTH release at lower antagonist:agonist ratios (3:1) compared to αhCRF(9-41) (3,000:1 intravenously) .
- Therapeutic Use : More effective than αhCRF(9-41) in reversing CRF-driven anxiety in rodent models .
Receptor Selectivity and Signaling Profiles
In Vivo Efficacy and Therapeutic Potential
- αhCRF(9-41): Central vs. Peripheral Effects: Intracerebroventricular (icv) administration effectively blocks CRF-induced plasma catecholamine release (antagonist:agonist ratio 6:1), while intravenous (iv) administration requires 3,000:1 to inhibit ACTH release, highlighting blood-brain barrier limitations . Fever Reduction: Synergizes with indomethacin to suppress endotoxin-induced fever in rabbits, suggesting combined therapeutic strategies .
Urocortin III :
Limitations and Controversies
- Partial Agonism : αhCRF(9-41)’s weak CRF1 agonism may confound antagonist studies, as observed in Cos7 cells . This property is absent in D-PheCRF(12-41) and astressin derivatives .
- Batch Variability : Inconsistent reports of partial agonism may arise from differences in peptide synthesis or receptor expression levels .
Biological Activity
Alpha-helical corticotropin-releasing factor (CRF) (9-41) is a peptide antagonist that plays a significant role in modulating the biological effects of corticotropin-releasing factor, a critical hormone involved in the stress response. This article explores the biological activity of alpha-helical CRF (9-41), detailing its mechanisms, effects on various physiological processes, and implications for therapeutic applications.
Overview of Corticotropin-Releasing Factor
Corticotropin-releasing factor is a 41-amino acid peptide that regulates the hypothalamic-pituitary-adrenal (HPA) axis, influencing stress responses, anxiety, and other neuroendocrine functions. Upon stress exposure, CRF is released from the hypothalamus, stimulating the secretion of adrenocorticotropic hormone (ACTH) from the anterior pituitary, which in turn promotes cortisol release from the adrenal glands .
Alpha-helical CRF (9-41) acts primarily as an antagonist at CRF receptors. Its affinity for different receptor subtypes is characterized by the following Ki values:
This differential affinity highlights its potential for selective modulation of CRF signaling pathways.
1. Anxiolytic Properties
Research indicates that alpha-helical CRF (9-41) can mitigate anxiety-related behaviors induced by various stimuli. For instance, in studies involving nicotine-induced anxiety responses in rats, alpha-helical CRF (9-41) effectively reversed conditioned anxiety without affecting unconditioned responses . This suggests its potential utility in anxiety disorders where CRF signaling is dysregulated.
2. Stress Response Modulation
Alpha-helical CRF (9-41) has been shown to blunt stress-induced alterations in various physiological parameters. In vivo studies demonstrate that this peptide can inhibit stress-induced increases in ACTH and cortisol levels, thereby modulating the HPA axis response to stressors .
3. Neuroendocrine Effects
The antagonist has been implicated in altering neuroendocrine functions beyond simple inhibition of ACTH release. For example, it has been observed to affect food intake and metabolic processes through its action on CRF receptors in specific brain regions .
Table 1: Summary of Key Studies on Alpha-Helical CRF (9-41)
Implications for Therapeutic Applications
Given its role as a selective antagonist of CRF receptors, alpha-helical CRF (9-41) holds promise for developing novel therapies targeting stress-related disorders such as anxiety and depression. By modulating the HPA axis and reducing excessive CRF signaling, it may help restore balance in patients suffering from chronic stress conditions.
Q & A
Basic Research Questions
Q. What is the primary mechanism of αhCRF (9-41) as a CRF antagonist, and how is this determined experimentally?
αhCRF (9-41) acts as a competitive antagonist at CRF2 receptors and a partial agonist at CRF1 receptors. This dual activity is identified using receptor binding assays (e.g., displacement studies with radiolabeled CRF) and functional assays measuring cAMP production or downstream signaling pathways. For example, in vivo studies in rats demonstrate differential antagonism depending on administration routes (intracerebroventricular vs. intravenous) .
Q. Which in vivo models are commonly used to study αhCRF (9-41)'s effects on stress and behavior?
- Stress-induced colitis models : Partial restraint stress in rats combined with intracolonic TNB instillation to evaluate colitis exacerbation .
- Acoustic startle response : CRF-induced potentiation of startle reflexes reversed by αhCRF (9-41) in rodents .
- Body fluid homeostasis : Bilateral LPBN injections to assess CRF's role in fluid regulation .
Q. How is αhCRF (9-41) purity and structural integrity validated for experimental use?
Analytical methods include:
- High-Performance Liquid Chromatography (HPLC) : Purity ≥97% confirmed via retention time and peak analysis .
- Mass spectrometry : Molecular weight verification (3220.66 Da) and amino acid sequencing .
Advanced Research Questions
Q. How do antagonist:agonist ratios vary across experimental systems, and how should researchers optimize dosing?
Antagonist efficacy depends on the bioassay system:
Q. How can researchers reconcile contradictory findings on αhCRF (9-41)'s role in stress-exacerbated colitis?
In rat colitis models, αhCRF (9-41) failed to inhibit TNB-induced inflammation but amplified stress-related colitis severity. This paradox may arise from tissue-specific CRF receptor subtypes or interactions with non-CRF pathways (e.g., arginine vasopressin). Researchers should:
- Use receptor subtype-selective antagonists (e.g., CRF1 vs. CRF2).
- Include stress-only and antagonist-only control groups .
Q. What methodological challenges arise when studying CRF receptor subtype specificity with αhCRF (9-41)?
- Partial agonism at CRF1 : May confound results in systems where CRF1 activation modulates outcomes.
- Cross-reactivity : Validate receptor interactions using CRISPR/Cas9 knockout models or selective ligands (e.g., Urocortin III for CRF2) .
Q. How do experimental designs account for CRF's dual role in endocrine and behavioral pathways?
- Multimodal endpoints : Combine plasma ACTH measurements (ELISA) with behavioral assays (e.g., acoustic startle, open-field tests) .
- Temporal profiling : Administer αhCRF (9-41) at stressor-specific timepoints (e.g., pre-stress vs. post-stress) to dissect acute vs. chronic effects .
Q. Data Contradiction Analysis
Q. Why does αhCRF (9-41) show differential efficacy in blocking CRF-induced ACTH vs. catecholamine release?
The 3000:1 ratio required for ACTH suppression suggests peripheral vs. central CRF receptor populations or pharmacokinetic barriers (e.g., blood-brain permeability). Researchers should:
- Compare central (e.g., CSF) and peripheral CRF levels via immunoassays.
- Use site-specific microinjections to isolate receptor subtypes .
Q. Methodological Recommendations
Properties
IUPAC Name |
methyl (2S)-2-[[(3R)-3-hydroxy-4-(phenylmethoxycarbonylamino)butanoyl]amino]-3-(2-iodophenyl)propanoate | |
---|---|---|
Details | Computed by Lexichem TK 2.7.0 (PubChem release 2021.05.07) | |
Source | PubChem | |
URL | https://pubchem.ncbi.nlm.nih.gov | |
Description | Data deposited in or computed by PubChem | |
InChI |
InChI=1S/C22H25IN2O6/c1-30-21(28)19(11-16-9-5-6-10-18(16)23)25-20(27)12-17(26)13-24-22(29)31-14-15-7-3-2-4-8-15/h2-10,17,19,26H,11-14H2,1H3,(H,24,29)(H,25,27)/t17-,19+/m1/s1 | |
Details | Computed by InChI 1.0.6 (PubChem release 2021.05.07) | |
Source | PubChem | |
URL | https://pubchem.ncbi.nlm.nih.gov | |
Description | Data deposited in or computed by PubChem | |
InChI Key |
QDUCLNZKQMUVKX-MJGOQNOKSA-N | |
Details | Computed by InChI 1.0.6 (PubChem release 2021.05.07) | |
Source | PubChem | |
URL | https://pubchem.ncbi.nlm.nih.gov | |
Description | Data deposited in or computed by PubChem | |
Canonical SMILES |
COC(=O)C(CC1=CC=CC=C1I)NC(=O)CC(CNC(=O)OCC2=CC=CC=C2)O | |
Details | Computed by OEChem 2.3.0 (PubChem release 2021.05.07) | |
Source | PubChem | |
URL | https://pubchem.ncbi.nlm.nih.gov | |
Description | Data deposited in or computed by PubChem | |
Isomeric SMILES |
COC(=O)[C@H](CC1=CC=CC=C1I)NC(=O)C[C@H](CNC(=O)OCC2=CC=CC=C2)O | |
Details | Computed by OEChem 2.3.0 (PubChem release 2021.05.07) | |
Source | PubChem | |
URL | https://pubchem.ncbi.nlm.nih.gov | |
Description | Data deposited in or computed by PubChem | |
Molecular Formula |
C22H25IN2O6 | |
Details | Computed by PubChem 2.1 (PubChem release 2021.05.07) | |
Source | PubChem | |
URL | https://pubchem.ncbi.nlm.nih.gov | |
Description | Data deposited in or computed by PubChem | |
Molecular Weight |
540.3 g/mol | |
Details | Computed by PubChem 2.1 (PubChem release 2021.05.07) | |
Source | PubChem | |
URL | https://pubchem.ncbi.nlm.nih.gov | |
Description | Data deposited in or computed by PubChem | |
Retrosynthesis Analysis
AI-Powered Synthesis Planning: Our tool employs the Template_relevance Pistachio, Template_relevance Bkms_metabolic, Template_relevance Pistachio_ringbreaker, Template_relevance Reaxys, Template_relevance Reaxys_biocatalysis model, leveraging a vast database of chemical reactions to predict feasible synthetic routes.
One-Step Synthesis Focus: Specifically designed for one-step synthesis, it provides concise and direct routes for your target compounds, streamlining the synthesis process.
Accurate Predictions: Utilizing the extensive PISTACHIO, BKMS_METABOLIC, PISTACHIO_RINGBREAKER, REAXYS, REAXYS_BIOCATALYSIS database, our tool offers high-accuracy predictions, reflecting the latest in chemical research and data.
Strategy Settings
Precursor scoring | Relevance Heuristic |
---|---|
Min. plausibility | 0.01 |
Model | Template_relevance |
Template Set | Pistachio/Bkms_metabolic/Pistachio_ringbreaker/Reaxys/Reaxys_biocatalysis |
Top-N result to add to graph | 6 |
Feasible Synthetic Routes
Disclaimer and Information on In-Vitro Research Products
Please be aware that all articles and product information presented on BenchChem are intended solely for informational purposes. The products available for purchase on BenchChem are specifically designed for in-vitro studies, which are conducted outside of living organisms. In-vitro studies, derived from the Latin term "in glass," involve experiments performed in controlled laboratory settings using cells or tissues. It is important to note that these products are not categorized as medicines or drugs, and they have not received approval from the FDA for the prevention, treatment, or cure of any medical condition, ailment, or disease. We must emphasize that any form of bodily introduction of these products into humans or animals is strictly prohibited by law. It is essential to adhere to these guidelines to ensure compliance with legal and ethical standards in research and experimentation.