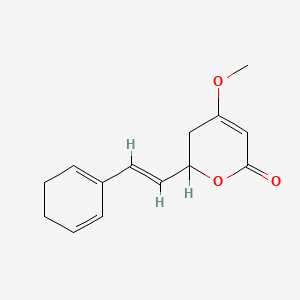
Kava
Overview
Description
Kava (Piper methysticum), a perennial shrub native to the Pacific Islands, has been consumed for centuries as a traditional beverage for its anxiolytic, sedative, and social-relaxation properties . Its bioactive constituents, primarily kavalactones and flavokavains, are responsible for its pharmacological effects. Kavalactones, a group of α-pyrone derivatives, include six major compounds: yangonin, methysticin, dihydromethysticin (DHM), dihydrokawain (DHK), kawain (K), and demethoxyyangonin . Flavokavains (FKA, FKB, FKC), chalcone-based compounds, are structurally distinct and linked to both therapeutic and hepatotoxic effects .
Preparation Methods
Synthetic Routes and Reaction Conditions: Kava is typically prepared from the root of the Piper methysticum plant. The traditional preparation method involves grinding the root into a fine powder, which is then mixed with water and strained to produce a beverage . Industrial production methods may involve more advanced techniques such as extraction and purification to isolate the active kavalactones .
Chemical Reactions Analysis
Metabolic Pathways of Kavalactones
Kavalactones are metabolized primarily via hepatic cytochrome P450 (CYP450) enzymes, with variations observed across species and human subjects. Key metabolic transformations include:
-
Demethylation : Removal of methoxy groups (e.g., 4-methoxy or 12-methoxy substituents) from the α-pyrone ring .
-
Hydroxylation : Addition of hydroxyl groups at C-12 positions, observed in yangonin and desmethoxyyangonin .
-
Reduction : Saturation of the 3,4-double bond in dihydrokavain and dihydromethysticin .
-
Ring-opening : Enzymatic cleavage of the lactone ring in compounds like 7,8-dihydromethysticin, forming mercapturic acid adducts .
Table 1: Major Human Metabolites of Kavalactones
Enzyme Inhibition and Drug Interactions
Kavalactones and flavokawains exhibit significant inhibition of CYP enzymes, impacting drug metabolism:
-
CYP2C9/CYP3A4 inhibition : Methysticin (IC<sub>50</sub> = 10 µM) and dihydromethysticin (IC<sub>50</sub> = 17 µM) reduce enzymatic activity by >50% .
-
CYP2D6 suppression : Flavokavain A inhibits CYP2D6 (73% activity reduction) .
Table 2: CYP Enzyme Inhibition by this compound Components
Compound | Inhibited Enzymes | % Inhibition (10 µM) |
---|---|---|
Methysticin | CYP2C9, CYP3A4 | 54–76% |
Flavokavain A | CYP2D6 | 73% |
Desmethoxyyangonin | CYP1A2 | 40% |
Formation of Reactive Metabolites
Oxidative metabolism generates electrophilic intermediates linked to hepatotoxicity:
-
Kavain 11,12-quinone : Formed via CYP-mediated oxidation, detected as a glutathione conjugate in human microsomes .
-
7,8-Dihydrokavain 11,12-quinone : A reactive metabolite implicated in mitochondrial toxicity .
These quinones deplete glutathione reserves and form protein adducts, triggering oxidative stress .
Extraction and Solvent Effects on Chemical Composition
Solvent polarity determines kavalactone yield and flavokawain content:
-
Acetone : Extracts 20–25% total kavalactones, highest among solvents .
-
Ethanol : Yields 15–18% kavalactones but increases flavokawain B by 100× vs. water .
-
Water : Traditional method; yields <5% kavalactones, minimal flavokawains .
Table 3: Solvent Efficiency in Kavalactone Extraction
Solvent | Total Kavalactones (%) | Flavokawain B (ppm) |
---|---|---|
Acetone | 20–25 | 120 |
Ethanol | 15–18 | 450 |
Water | 3–5 | 5 |
This synthesis underscores the interplay between this compound’s chemistry and its biological effects, emphasizing the need for standardized extraction protocols and metabolic safety assessments .
Scientific Research Applications
Chemical Composition and Mechanisms of Action
Kava contains a unique group of compounds known as kavalactones, which are primarily responsible for its psychoactive effects. The main kavalactones include:
- Kavain
- Dihydrokavain
- Yangonin
- Desmethoxyyangonin
These compounds interact with various neurotransmitter systems in the brain, particularly GABA receptors, which are crucial for anxiety modulation. Research indicates that this compound may enhance GABAergic activity, leading to its anxiolytic effects .
Anxiety Management
This compound has been widely studied for its efficacy in treating anxiety disorders. Meta-analyses have shown that this compound extracts can significantly reduce anxiety symptoms in patients with generalized anxiety disorder (GAD) and chronic anxiety .
Anti-inflammatory Effects
Recent studies suggest that this compound and its constituents exhibit anti-inflammatory properties. These effects may be beneficial in treating conditions characterized by inflammation, such as arthritis and other inflammatory diseases .
Potential Anticancer Properties
Emerging research points to this compound's potential role in cancer prevention and treatment. Some studies have indicated that kavalactones may inhibit the proliferation of cancer cells and induce apoptosis (programmed cell death) in certain cancer types . However, further investigation is necessary to confirm these findings.
Safety and Toxicity Concerns
Despite its therapeutic potential, this compound has been associated with hepatotoxicity in some cases. Reports of liver damage have raised concerns about its safety profile, particularly with unregulated or high-dose consumption . The FDA has issued warnings regarding this compound products due to these risks, emphasizing the need for quality control and standardized dosing .
Case Studies
Case Study 1: Efficacy in Generalized Anxiety Disorder
A randomized controlled trial involving 60 participants demonstrated that those receiving this compound extract showed a significant reduction in anxiety levels compared to a placebo group over a period of four weeks. The study utilized a standardized this compound extract containing 70% kavalactones .
Case Study 2: Anti-inflammatory Effects
In a double-blind study assessing the effects of this compound on patients with chronic pain conditions, participants reported a notable decrease in inflammation markers after eight weeks of treatment with a specific this compound extract formulation .
Summary of Research Findings
Application | Evidence Level | Key Findings |
---|---|---|
Anxiety Management | Strong | Significant reduction in anxiety symptoms; effective for chronic anxiety |
Anti-inflammatory Effects | Moderate | Potential benefits for inflammatory conditions; requires further research |
Anticancer Properties | Emerging | Inhibition of cancer cell proliferation observed; more studies needed |
Safety Concerns | High | Reports of hepatotoxicity; need for standardization and quality control |
Mechanism of Action
The primary mechanism of action of kava involves the modulation of gamma-aminobutyric acid type A (GABA-A) receptors in the brain . Kavalactones enhance GABAergic neurotransmission, leading to increased inhibitory effects and resulting in the calming and relaxing properties of this compound . Additionally, kavalactones inhibit monoamine oxidases, which are enzymes involved in the breakdown of neurotransmitters such as serotonin and dopamine .
Comparison with Similar Compounds
Chemical Composition and Key Compounds
Kava’s chemical diversity arises from over 150 cultivars, extraction methods (aqueous vs. ethanolic), and plant parts used . Key compounds include:
Kavalactones vs. Flavokavains
While both classes derive from the same biosynthetic pathway, their biological profiles diverge:
Aqueous vs. Ethanolic Extracts
Solvent choice critically impacts compound extraction and safety:
Whole Extract vs. Isolated Compounds
Synergistic effects of the whole extract contrast with isolated compounds:
- Kavalactones in Isolation : Lack the full anxiolytic and neuroprotective efficacy of the whole extract .
- Flavokavains in Isolation : Exhibit cytotoxicity (e.g., FKA/FKB induce liver damage in mice) but lack the anti-inflammatory benefits of kavalactone mixtures .
- Polypharmacological Advantage: Whole extracts provide multi-target benefits (e.g., carcinogen detoxification, stress reduction) unmatched by single compounds .
Research Findings and Clinical Implications
- Anxiety Relief : Meta-analyses confirm this compound extract’s superiority over placebo (Hamilton Anxiety Score reduction: 9.7 points) with mild adverse effects .
Biological Activity
Kava, derived from the root of the plant Piper methysticum, has been utilized for centuries in Pacific Island cultures for its psychoactive properties. The primary bioactive compounds in this compound are kavalactones and flavokawains, which exhibit various biological activities, including anxiolytic, antioxidant, and anticancer effects. However, concerns regarding hepatotoxicity have emerged, necessitating a comprehensive review of its biological activity.
1. Chemical Composition of this compound
This compound's biological activity is largely attributed to its chemical constituents:
-
Kavalactones: These are the most studied compounds in this compound, with six major kavalactones accounting for 20–50% of the dry weight of this compound root. They include:
- Kawain
- Dihydrokawain
- Yangonin
- Methysticin
- Desmethoxyyangonin
- Kavain
- Flavokawains: These compounds are less prevalent but have demonstrated significant biological effects, particularly in anticancer studies.
Compound | Percentage in this compound Root | Biological Activity |
---|---|---|
Kavalactones | 20-50% | Anxiolytic, Antioxidant |
Flavokawains | Varies | Anticancer |
2.1 Anxiolytic Effects
This compound has been shown to possess anxiolytic properties comparable to benzodiazepines. A systematic review indicated that kavalactones can modulate GABAergic neurotransmission, leading to reduced anxiety without the sedative effects typical of traditional anxiolytics .
2.2 Antioxidant Properties
Research has demonstrated that this compound extracts exhibit strong antioxidant activity, which can help mitigate oxidative stress and prevent cellular damage. Specific flavonoids isolated from this compound have been identified as potent antioxidants .
2.3 Anticancer Potential
Kavalactones and flavokawains have shown promising results in preclinical studies for their ability to inhibit cancer cell proliferation and induce apoptosis in various cancer cell lines. For example, flavokawains have been linked to significant anticancer activity against breast and prostate cancer cells .
3. Hepatotoxicity Concerns
Despite its beneficial effects, this compound consumption has been associated with hepatotoxicity. Clinical reports suggest that certain compounds in this compound, particularly flavokawains, may contribute to liver damage when consumed in high quantities or over extended periods .
Case Studies on Hepatotoxicity:
- A systematic review highlighted cases where individuals consuming high doses of this compound experienced liver dysfunction, prompting regulatory scrutiny in several countries .
- Another study indicated that while kavalactones showed minimal hepatotoxicity in animal models, flavokawains exhibited significant cytotoxic effects in vitro .
4. Cognitive Effects and Driving Safety
Recent studies have investigated the cognitive effects of this compound consumption, particularly concerning driving safety. A study involving participants consuming traditional volumes of this compound (up to 3.52 liters) found no significant impairment in reaction times or divided attention compared to a control group . This suggests that while this compound does produce sedative effects, it may not significantly impair cognitive functions at traditionally consumed levels.
5. Future Research Directions
Given the diverse biological activities and potential risks associated with this compound consumption, further research is essential:
- Mechanistic Studies: Investigating the precise mechanisms through which kavalactones and flavokawains exert their biological effects.
- Longitudinal Studies: Assessing the long-term impacts of regular this compound consumption on liver health and cognitive function.
- Quality Control: Establishing standards for this compound preparation and consumption to minimize risks associated with hepatotoxicity.
Q & A
Basic Research Questions
Q. How should researchers standardize kava extracts for pharmacological studies to ensure reproducibility?
- Methodological Guidance : Use high-performance liquid chromatography (HPLC) or gas chromatography (GC) to quantify kavalactones (e.g., kavain, methysticin) and establish batch-specific chemical profiles. Reference cultivar authentication via genetic markers or geographical sourcing to control variability. Include solvent type (aqueous vs. acetonic) and extraction duration in protocols, as these affect bioactive compound ratios .
- Data Example :
Kavalactone | Concentration Range (mg/g) | Solvent Used |
---|---|---|
Kavain | 10–45 | Water |
Methysticin | 8–32 | Ethanol |
Q. What experimental designs are recommended for assessing this compound’s anxiolytic effects in clinical trials?
- Methodological Guidance : Employ randomized, double-blind, placebo-controlled trials (RCTs) with validated scales (e.g., Hamilton Anxiety Rating Scale). Control for confounding variables like concomitant medication use and participant ethnicity, as genetic polymorphisms may influence kavalactone metabolism. Use standardized extracts (e.g., WS® 1490) with documented kavalactone content .
Q. How can researchers address contradictory findings on this compound-induced hepatotoxicity?
- Methodological Guidance : Conduct systematic reviews with meta-analyses to differentiate between studies using traditional aqueous preparations (low risk) and ethanol-based extracts (higher risk). Include liver function biomarkers (ALT, AST) in trial protocols and stratify data by preparation method, dosage, and duration .
Advanced Research Questions
Q. What strategies resolve discrepancies in pharmacokinetic data for kavalactones across in vitro and in vivo models?
- Methodological Guidance : Use physiologically based pharmacokinetic (PBPK) modeling to account for interspecies differences. Validate models with human clinical data, prioritizing cohorts with traditional this compound consumption histories. Analyze cytochrome P450 enzyme interactions (e.g., CYP2E1 inhibition) to clarify metabolic pathways .
Q. How can ethnopharmacological studies on traditional this compound use be structured to ensure methodological rigor?
- Methodological Guidance : Apply mixed-methods frameworks combining qualitative interviews (e.g., Indigenous knowledge documentation) with quantitative analysis of preparation techniques. Use participatory research models to align study objectives with community priorities. Standardize ethnographic data collection via structured observation protocols .
Q. What approaches validate this compound’s potential as a muscle relaxant or sleep aid, given limited clinical data?
- Methodological Guidance : Design preclinical studies using electromyography (EMG) to measure muscle activity in animal models. For sleep research, employ polysomnography to assess sleep architecture (REM, NREM cycles) in human trials. Prioritize isolating specific kavalactones (e.g., yangonin) to identify mechanistic targets .
Q. How should researchers mitigate microbiological risks in this compound beverage studies while preserving traditional preparation methods?
- Methodological Guidance : Integrate Good Agricultural Practices (GAP) and Good Hygienic Practices (GHP) during root harvesting and processing. Conduct microbial load assays (e.g., Salmonella spp., E. coli) on raw materials and finished products. Compare traditional fermentation practices with controlled laboratory methods to balance safety and cultural authenticity .
Q. Data Analysis & Replication Challenges
Q. What statistical methods are optimal for analyzing dose-response relationships in this compound toxicity studies?
- Methodological Guidance : Apply benchmark dose (BMD) modeling to estimate safe exposure levels. Use multivariate regression to adjust for covariates like body mass index (BMI) and liver health status. Share raw data via open-access repositories to facilitate reproducibility .
Q. How can researchers improve replicability in studies measuring this compound’s effects on human balance or motor function?
- Methodological Guidance : Adopt motion-capture technology to quantify postural sway with high precision. Standardize testing conditions (e.g., fasting state, ambient noise) and publish detailed protocols, as seen in body sway studies .
Q. Tables for Reference
Table 1 : Key kavalactones and their proposed mechanisms
Kavalactone | Primary Mechanism | Bioactivity Range |
---|---|---|
Kavain | GABA receptor modulation | 50–200 mg/day |
Methysticin | Dopamine reuptake inhibition | 20–100 mg/day |
Table 2 : Common pitfalls in this compound clinical trials and solutions
Pitfall | Solution |
---|---|
Unstandardized extracts | Use certified batches with HPLC profiles |
Ignoring preparation methods | Stratify data by solvent type (water vs. ethanol) |
Properties
IUPAC Name |
2-[(E)-2-cyclohexa-1,5-dien-1-ylethenyl]-4-methoxy-2,3-dihydropyran-6-one | |
---|---|---|
Details | Computed by Lexichem TK 2.7.0 (PubChem release 2021.05.07) | |
Source | PubChem | |
URL | https://pubchem.ncbi.nlm.nih.gov | |
Description | Data deposited in or computed by PubChem | |
InChI |
InChI=1S/C14H16O3/c1-16-13-9-12(17-14(15)10-13)8-7-11-5-3-2-4-6-11/h3,5-8,10,12H,2,4,9H2,1H3/b8-7+ | |
Details | Computed by InChI 1.0.6 (PubChem release 2021.05.07) | |
Source | PubChem | |
URL | https://pubchem.ncbi.nlm.nih.gov | |
Description | Data deposited in or computed by PubChem | |
InChI Key |
OMNGEVNATYFZGG-BQYQJAHWSA-N | |
Details | Computed by InChI 1.0.6 (PubChem release 2021.05.07) | |
Source | PubChem | |
URL | https://pubchem.ncbi.nlm.nih.gov | |
Description | Data deposited in or computed by PubChem | |
Canonical SMILES |
COC1=CC(=O)OC(C1)C=CC2=CCCC=C2 | |
Details | Computed by OEChem 2.3.0 (PubChem release 2021.05.07) | |
Source | PubChem | |
URL | https://pubchem.ncbi.nlm.nih.gov | |
Description | Data deposited in or computed by PubChem | |
Isomeric SMILES |
COC1=CC(=O)OC(C1)/C=C/C2=CCCC=C2 | |
Details | Computed by OEChem 2.3.0 (PubChem release 2021.05.07) | |
Source | PubChem | |
URL | https://pubchem.ncbi.nlm.nih.gov | |
Description | Data deposited in or computed by PubChem | |
Molecular Formula |
C14H16O3 | |
Details | Computed by PubChem 2.1 (PubChem release 2021.05.07) | |
Source | PubChem | |
URL | https://pubchem.ncbi.nlm.nih.gov | |
Description | Data deposited in or computed by PubChem | |
Molecular Weight |
232.27 g/mol | |
Details | Computed by PubChem 2.1 (PubChem release 2021.05.07) | |
Source | PubChem | |
URL | https://pubchem.ncbi.nlm.nih.gov | |
Description | Data deposited in or computed by PubChem | |
CAS No. |
9000-38-8 | |
Record name | Kava | |
Source | DrugBank | |
URL | https://www.drugbank.ca/drugs/DB01322 | |
Description | The DrugBank database is a unique bioinformatics and cheminformatics resource that combines detailed drug (i.e. chemical, pharmacological and pharmaceutical) data with comprehensive drug target (i.e. sequence, structure, and pathway) information. | |
Explanation | Creative Common's Attribution-NonCommercial 4.0 International License (http://creativecommons.org/licenses/by-nc/4.0/legalcode) | |
Retrosynthesis Analysis
AI-Powered Synthesis Planning: Our tool employs the Template_relevance Pistachio, Template_relevance Bkms_metabolic, Template_relevance Pistachio_ringbreaker, Template_relevance Reaxys, Template_relevance Reaxys_biocatalysis model, leveraging a vast database of chemical reactions to predict feasible synthetic routes.
One-Step Synthesis Focus: Specifically designed for one-step synthesis, it provides concise and direct routes for your target compounds, streamlining the synthesis process.
Accurate Predictions: Utilizing the extensive PISTACHIO, BKMS_METABOLIC, PISTACHIO_RINGBREAKER, REAXYS, REAXYS_BIOCATALYSIS database, our tool offers high-accuracy predictions, reflecting the latest in chemical research and data.
Strategy Settings
Precursor scoring | Relevance Heuristic |
---|---|
Min. plausibility | 0.01 |
Model | Template_relevance |
Template Set | Pistachio/Bkms_metabolic/Pistachio_ringbreaker/Reaxys/Reaxys_biocatalysis |
Top-N result to add to graph | 6 |
Feasible Synthetic Routes
Disclaimer and Information on In-Vitro Research Products
Please be aware that all articles and product information presented on BenchChem are intended solely for informational purposes. The products available for purchase on BenchChem are specifically designed for in-vitro studies, which are conducted outside of living organisms. In-vitro studies, derived from the Latin term "in glass," involve experiments performed in controlled laboratory settings using cells or tissues. It is important to note that these products are not categorized as medicines or drugs, and they have not received approval from the FDA for the prevention, treatment, or cure of any medical condition, ailment, or disease. We must emphasize that any form of bodily introduction of these products into humans or animals is strictly prohibited by law. It is essential to adhere to these guidelines to ensure compliance with legal and ethical standards in research and experimentation.