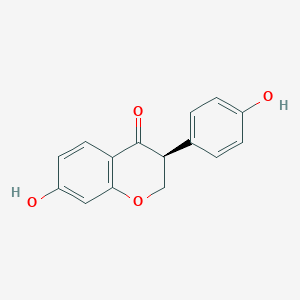
s-Dihydrodaidzein
Overview
Description
S-Dihydrodaidzein: is a metabolite of the isoflavone daidzein, which is found in soy products. It is produced by the action of gut bacteria and plays a crucial role in the biosynthesis of S-equol, a compound with high estrogen-like activity. This compound has garnered significant attention due to its potential health benefits, particularly in relation to cardiovascular health and menopausal symptom relief .
Scientific Research Applications
S-Dihydrodaidzein has several scientific research applications, including:
Chemistry: Used as an intermediate in the synthesis of S-equol, a compound with significant estrogenic activity.
Biology: Studied for its role in the gut microbiota and its impact on human health.
Medicine: Investigated for its potential benefits in cardiovascular health and menopausal symptom relief.
Industry: Utilized in the production of dietary supplements and functional foods.
Mechanism of Action
Target of Action
DihydroDaidzein (DHD) is an active metabolite of daidzein . It primarily targets estrogen receptors, exhibiting estrogenic activity . The compound’s primary targets are therefore the estrogen receptors, which play a crucial role in various biological processes, including the regulation of the menstrual cycle and reproductive development.
Mode of Action
DHD interacts with its targets, the estrogen receptors, by binding to them . This binding stimulates the estrogen receptor-dependent growth of cells, such as breast cancer MCF-7 cells, at micromolar concentrations . The interaction with its targets results in changes at the cellular level, influencing cell growth and proliferation.
Biochemical Pathways
DHD is primarily derived from the principal constituents of soy isoflavones (SIF)—daidzein (DAI), through hydrogenation in metabolism . The intestinal microbiota plays a pivotal role in the biotransformation of DAI, and the enrichment and substantial synthesis of DHD depend on the selection of highly efficient bacterial strains from the multitude participating in DAI conversion .
Pharmacokinetics
The pharmacokinetic properties of DHD are closely related to those of its precursor, daidzein
Result of Action
The action of DHD results in various molecular and cellular effects. It has been found to have vasodilatory action on rat isolated aortic rings . Furthermore, it stimulates the estrogen receptor-dependent growth of breast cancer MCF-7 cells at micromolar concentrations .
Action Environment
The action, efficacy, and stability of DHD can be influenced by various environmental factors. For instance, the metabolism of daidzein to DHD is significantly influenced by the presence and composition of intestinal bacteria . .
Safety and Hazards
Future Directions
Biochemical Analysis
Biochemical Properties
DHD interacts with various enzymes, proteins, and other biomolecules. It is produced by the anaerobic bioconversion of isoflavones daidzein and genistein by certain bacterial strains . The conversion of daidzein to DHD is primarily achieved through the secretion of a series of enzymes . The bioconversion rate of daidzein by certain bacterial strains can reach up to 60.3% .
Cellular Effects
DHD has been found to have significant effects on various types of cells and cellular processes. It has been reported to exhibit anti-inflammatory activities and inhibitory effects on human vascular smooth muscle cell proliferation . DHD is also known to influence cell function, including impacts on cell signaling pathways, gene expression, and cellular metabolism .
Molecular Mechanism
The molecular mechanism of DHD involves its interactions with biomolecules at the molecular level. It exerts its effects through binding interactions with biomolecules, enzyme inhibition or activation, and changes in gene expression . For instance, DHD is known to interact with mitogen-activated protein kinase 8 (JNK1), which is consistent with its anti-inflammatory activities .
Metabolic Pathways
DHD is involved in several metabolic pathways. It is primarily derived from the principal constituents of soy isoflavones—daidzein—through hydrogenation in metabolism . The metabolic pathways of daidzein by intestinal bacteria involve deglycosylation, hydroxylation, methoxylation, and acetylation .
Preparation Methods
Synthetic Routes and Reaction Conditions: The synthesis of S-Dihydrodaidzein typically involves the reduction of daidzein. This can be achieved using daidzein reductase enzymes, which convert daidzein to dihydrodaidzein under anaerobic conditions. The reaction conditions often require specific cofactors and a controlled environment to ensure the desired enantioselectivity .
Industrial Production Methods: Industrial production of this compound can be achieved through bioconversion processes using engineered strains of bacteria such as Escherichia coli. These strains are modified to express the necessary enzymes for the conversion of daidzein to this compound. The process involves optimizing reaction conditions, such as pH and temperature, to maximize yield and productivity .
Chemical Reactions Analysis
Types of Reactions: S-Dihydrodaidzein undergoes several types of chemical reactions, including:
Reduction: Conversion to tetrahydrodaidzein using dihydrodaidzein reductase.
Oxidation: Reversible conversion back to daidzein under aerobic conditions.
Common Reagents and Conditions:
Reduction: Requires dihydrodaidzein reductase and NADPH as a cofactor.
Oxidation: Can occur without cofactors under aerobic conditions.
Major Products:
Reduction: Tetrahydrodaidzein.
Oxidation: Daidzein.
Comparison with Similar Compounds
Daidzein: The precursor to S-Dihydrodaidzein, found in soy products.
Genistein: Another isoflavone with estrogenic activity.
Uniqueness: this compound is unique due to its specific role in the biosynthesis of S-equol, which has higher estrogenic activity compared to other isoflavones. This makes it particularly valuable for its health benefits and potential therapeutic applications .
Properties
IUPAC Name |
(3S)-7-hydroxy-3-(4-hydroxyphenyl)-2,3-dihydrochromen-4-one | |
---|---|---|
Details | Computed by LexiChem 2.6.6 (PubChem release 2019.06.18) | |
Source | PubChem | |
URL | https://pubchem.ncbi.nlm.nih.gov | |
Description | Data deposited in or computed by PubChem | |
InChI |
InChI=1S/C15H12O4/c16-10-3-1-9(2-4-10)13-8-19-14-7-11(17)5-6-12(14)15(13)18/h1-7,13,16-17H,8H2/t13-/m1/s1 | |
Details | Computed by InChI 1.0.5 (PubChem release 2019.06.18) | |
Source | PubChem | |
URL | https://pubchem.ncbi.nlm.nih.gov | |
Description | Data deposited in or computed by PubChem | |
InChI Key |
JHYXBPPMXZIHKG-CYBMUJFWSA-N | |
Details | Computed by InChI 1.0.5 (PubChem release 2019.06.18) | |
Source | PubChem | |
URL | https://pubchem.ncbi.nlm.nih.gov | |
Description | Data deposited in or computed by PubChem | |
Canonical SMILES |
C1C(C(=O)C2=C(O1)C=C(C=C2)O)C3=CC=C(C=C3)O | |
Details | Computed by OEChem 2.1.5 (PubChem release 2019.06.18) | |
Source | PubChem | |
URL | https://pubchem.ncbi.nlm.nih.gov | |
Description | Data deposited in or computed by PubChem | |
Isomeric SMILES |
C1[C@@H](C(=O)C2=C(O1)C=C(C=C2)O)C3=CC=C(C=C3)O | |
Details | Computed by OEChem 2.1.5 (PubChem release 2019.06.18) | |
Source | PubChem | |
URL | https://pubchem.ncbi.nlm.nih.gov | |
Description | Data deposited in or computed by PubChem | |
Molecular Formula |
C15H12O4 | |
Details | Computed by PubChem 2.1 (PubChem release 2019.06.18) | |
Source | PubChem | |
URL | https://pubchem.ncbi.nlm.nih.gov | |
Description | Data deposited in or computed by PubChem | |
Molecular Weight |
256.25 g/mol | |
Details | Computed by PubChem 2.1 (PubChem release 2021.05.07) | |
Source | PubChem | |
URL | https://pubchem.ncbi.nlm.nih.gov | |
Description | Data deposited in or computed by PubChem | |
Retrosynthesis Analysis
AI-Powered Synthesis Planning: Our tool employs the Template_relevance Pistachio, Template_relevance Bkms_metabolic, Template_relevance Pistachio_ringbreaker, Template_relevance Reaxys, Template_relevance Reaxys_biocatalysis model, leveraging a vast database of chemical reactions to predict feasible synthetic routes.
One-Step Synthesis Focus: Specifically designed for one-step synthesis, it provides concise and direct routes for your target compounds, streamlining the synthesis process.
Accurate Predictions: Utilizing the extensive PISTACHIO, BKMS_METABOLIC, PISTACHIO_RINGBREAKER, REAXYS, REAXYS_BIOCATALYSIS database, our tool offers high-accuracy predictions, reflecting the latest in chemical research and data.
Strategy Settings
Precursor scoring | Relevance Heuristic |
---|---|
Min. plausibility | 0.01 |
Model | Template_relevance |
Template Set | Pistachio/Bkms_metabolic/Pistachio_ringbreaker/Reaxys/Reaxys_biocatalysis |
Top-N result to add to graph | 6 |
Feasible Synthetic Routes
Q1: How is dihydrodaidzein formed in the body?
A1: Dihydrodaidzein is formed through the reduction of the isoflavone daidzein by specific bacteria residing in the human gut. [, , ]
Q2: Is dihydrodaidzein absorbed differently than daidzein?
A2: Research suggests that DHD, along with its analog dihydrogenistein, is absorbed more readily than their parent isoflavones, daidzein and genistein, respectively. This enhanced permeability might be attributed to their limited efflux transport and reduced glucuronidation/sulfation during intestinal transit. []
Q3: What evidence suggests that the small intestine plays a role in dihydrodaidzein metabolism?
A3: Studies using ileostomy subjects revealed the presence of DHD in their urine, albeit in lower amounts than in control subjects with intact gastrointestinal tracts. This finding suggests that while the small intestine contributes to DHD production, the large intestine plays a more significant role. []
Q4: Are there individual differences in the ability to produce dihydrodaidzein?
A5: Yes, similar to equol production, there are interindividual differences in the ability to produce DHD. In vitro fecal incubation studies revealed that not all individuals classified as equol non-producers could produce DHD, highlighting the variability in gut microbial composition and activity. [, ]
Q5: What are the potential health benefits associated with dihydrodaidzein?
A6: In vitro and in vivo studies suggest that DHD possesses anti-osteoporotic effects. In a mouse model of osteoporosis, DHD increased bone mineral density, potentially by upregulating bone morphogenic protein 2 (BMP2) and its downstream target osteopontin. Furthermore, DHD inhibited osteoclastogenesis in RAW264.7 cells by suppressing NF-κB activation and MAPK phosphorylation. []
Q6: Does dihydrodaidzein interact with estrogen receptors?
A7: While DHD itself is not a strong estrogen receptor agonist, it serves as a precursor to equol, a potent estrogen receptor beta (ERβ) ligand. [, ]
Q7: Does dihydrodaidzein affect adipogenesis?
A8: Yes, one study identified the S-enantiomer of DHD as a potential ERβ ligand exhibiting anti-adipogenic activity. In 3T3-L1 cells, S-dihydrodaidzein inhibited adipocyte differentiation, reduced lipid accumulation, and downregulated the expression of adipogenic markers PPARγ and C/EBPα. []
Q8: Does dihydrodaidzein have antioxidant activity?
A9: While DHD may possess some inherent antioxidant properties, its potential to be further metabolized into equol, a known antioxidant, is a significant aspect of its biological activity. [, ]
Q9: Which bacteria are known to produce dihydrodaidzein?
A10: Several bacterial species isolated from the human and animal gut have been shown to produce DHD, including Slackia isoflavoniconvertens, Adlercreutzia equolifaciens, Asaccharobacter celatus, Lactococcus sp. strain 20-92, and Clostridium-like bacterium strain TM-40. [, , , , , , , , , , ]
Q10: What enzymes are involved in the conversion of daidzein to dihydrodaidzein?
A11: The key enzyme responsible for this conversion is daidzein reductase (DZNR), which catalyzes the reduction of daidzein to DHD. [, ]
Q11: Can the production of dihydrodaidzein be enhanced?
A12: Studies have shown that the addition of specific nutrients, such as butyric acid and arginine, to bacterial cultures can enhance the conversion of daidzein to DHD. [] Additionally, genetic engineering approaches, such as cloning and heterologous expression of equol biosynthesis genes (including those involved in DHD production) in suitable hosts like Escherichia coli, are being explored for enhanced DHD and equol production. []
Q12: What analytical techniques are used to measure dihydrodaidzein levels?
A13: High-performance liquid chromatography (HPLC) coupled with various detection methods, such as mass spectrometry (MS) or ultraviolet (UV) detection, is commonly used for DHD quantification in biological samples like serum, urine, and fecal matter. [, , , , ]
Q13: Are there methods to detect and quantify the bacteria responsible for dihydrodaidzein production?
A14: Yes, quantitative PCR (qPCR) methods targeting specific genes involved in equol biosynthesis, such as dihydrodaidzein reductase (ddr) and tetrahydrodaidzein reductase (tdr), have been developed and used to detect and quantify equol-producing bacteria, including those capable of DHD production, in fecal samples. [, ]
Disclaimer and Information on In-Vitro Research Products
Please be aware that all articles and product information presented on BenchChem are intended solely for informational purposes. The products available for purchase on BenchChem are specifically designed for in-vitro studies, which are conducted outside of living organisms. In-vitro studies, derived from the Latin term "in glass," involve experiments performed in controlled laboratory settings using cells or tissues. It is important to note that these products are not categorized as medicines or drugs, and they have not received approval from the FDA for the prevention, treatment, or cure of any medical condition, ailment, or disease. We must emphasize that any form of bodily introduction of these products into humans or animals is strictly prohibited by law. It is essential to adhere to these guidelines to ensure compliance with legal and ethical standards in research and experimentation.