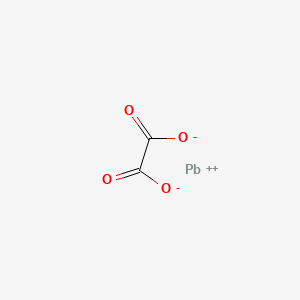
Lead oxalate
Overview
Description
Lead oxalate, with the chemical formula PbC₂O₄, is an organic compound that appears as a heavy white solid. It is naturally found in some minerals and can be synthesized in the laboratory. This compound is known for its low solubility in water and its role in various chemical processes and industrial applications.
Mechanism of Action
Target of Action
Lead oxalate primarily targets calcium ions in the body . It acts as a chelating agent, binding with calcium ions to form insoluble precipitates of calcium oxalates . This interaction with calcium ions is significant as it can influence various biological processes that rely on calcium.
Mode of Action
This compound interacts with its targets by forming insoluble precipitates . When this compound comes into contact with calcium ions, it binds with them to form these precipitates . This process is facilitated by the chelating ability of oxalates, which allows them to bind with divalent metals such as calcium .
Biochemical Pathways
The biochemical pathways affected by this compound involve the metabolism of oxalic acid . Oxalic acid is produced by fungi and bacteria and is involved in various processes such as nutrient availability, weathering of minerals, and precipitation of metal oxalates . The degradation of oxalic acid via decarboxylation is typical for fungi and is catalyzed by a lyase named oxalate decarboxylase . The activity of this enzyme leads to the decarboxylation of oxalic acid to formic acid and carbon dioxide .
Pharmacokinetics
It is known that this compound isinsoluble in water . Its solubility is increased in the presence of excess oxalate anions, due to the formation of the Pb(C2O4)2− complex ion . This suggests that the bioavailability of this compound may be influenced by the presence of other oxalate compounds in the body.
Result of Action
The molecular and cellular effects of this compound’s action are primarily related to its interaction with calcium ions. The formation of insoluble calcium oxalates can lead to the precipitation of these compounds in various tissues, which can cause damage . For example, the kidney is a primary target of oxalate toxicity . Elevated plasma concentrations and tissue accumulation of oxalate, which can occur due to the presence of this compound, are toxic to the body .
Preparation Methods
Synthetic Routes and Reaction Conditions: Lead oxalate can be synthesized through the reaction of lead nitrate (Pb(NO₃)₂) with oxalic acid (H₂C₂O₄). The reaction typically occurs in an aqueous solution, where lead nitrate and oxalic acid are mixed, resulting in the precipitation of this compound: [ \text{Pb(NO}_3\text{)}_2 + \text{H}_2\text{C}_2\text{O}_4 \rightarrow \text{PbC}_2\text{O}_4 + 2\text{HNO}_3 ]
Industrial Production Methods: In industrial settings, this compound is often produced as a byproduct of lead-acid battery recycling. The spent lead paste from batteries is treated with oxalic acid or sodium oxalate to precipitate this compound, which can then be further processed to produce lead oxide for new battery pastes .
Chemical Reactions Analysis
Types of Reactions: Lead oxalate undergoes various chemical reactions, including:
-
Thermal Decomposition: When heated, this compound decomposes to form lead oxide (PbO) and carbon dioxide (CO₂). [ \text{PbC}_2\text{O}_4 \rightarrow \text{PbO} + 2\text{CO}_2 ]
-
Reduction: this compound can be reduced to metallic lead (Pb) using reducing agents such as hydrogen gas (H₂) or carbon monoxide (CO). [ \text{PbC}_2\text{O}_4 + 2\text{H}_2 \rightarrow \text{Pb} + 2\text{CO}_2 + 2\text{H}_2\text{O} ]
Common Reagents and Conditions:
Oxalic Acid: Used in the synthesis of this compound.
Hydrogen Gas or Carbon Monoxide: Used in reduction reactions to produce metallic lead.
Major Products Formed:
Lead Oxide (PbO): Formed during thermal decomposition.
Metallic Lead (Pb): Formed during reduction reactions.
Scientific Research Applications
Lead oxalate has several scientific research applications, including:
Bioremediation: this compound can be transformed into less toxic compounds by microorganisms, making it useful in the bioremediation of lead-contaminated soils.
Material Science: this compound is used as a precursor for the synthesis of lead oxide nanoparticles, which have applications in batteries, sensors, and other electronic devices.
Analytical Chemistry: this compound is used in the quantification of oxalate ions in various samples.
Comparison with Similar Compounds
Lead oxalate can be compared with other metal oxalates, such as:
Calcium Oxalate (CaC₂O₄): Commonly found in kidney stones and plants.
Zinc Oxalate (ZnC₂O₄): Used in the synthesis of zinc oxide nanoparticles.
Cadmium Oxalate (CdC₂O₄): Known for its negative linear compressibility properties.
Uniqueness of this compound: this compound is unique due to its high density, low solubility, and its ability to be transformed into various lead compounds through thermal and chemical processes. Its role in bioremediation and material science further highlights its versatility and importance in scientific research.
Properties
IUPAC Name |
lead(2+);oxalate | |
---|---|---|
Details | Computed by Lexichem TK 2.7.0 (PubChem release 2021.05.07) | |
Source | PubChem | |
URL | https://pubchem.ncbi.nlm.nih.gov | |
Description | Data deposited in or computed by PubChem | |
InChI |
InChI=1S/C2H2O4.Pb/c3-1(4)2(5)6;/h(H,3,4)(H,5,6);/q;+2/p-2 | |
Details | Computed by InChI 1.0.6 (PubChem release 2021.05.07) | |
Source | PubChem | |
URL | https://pubchem.ncbi.nlm.nih.gov | |
Description | Data deposited in or computed by PubChem | |
InChI Key |
FCHAMWMIYDDXFS-UHFFFAOYSA-L | |
Details | Computed by InChI 1.0.6 (PubChem release 2021.05.07) | |
Source | PubChem | |
URL | https://pubchem.ncbi.nlm.nih.gov | |
Description | Data deposited in or computed by PubChem | |
Canonical SMILES |
C(=O)(C(=O)[O-])[O-].[Pb+2] | |
Details | Computed by OEChem 2.3.0 (PubChem release 2021.05.07) | |
Source | PubChem | |
URL | https://pubchem.ncbi.nlm.nih.gov | |
Description | Data deposited in or computed by PubChem | |
Molecular Formula |
PbC2O4, C2O4Pb | |
Details | Computed by PubChem 2.1 (PubChem release 2021.05.07) | |
Record name | lead oxalate | |
Source | Wikipedia | |
URL | https://en.wikipedia.org/wiki/Lead_oxalate | |
Description | Chemical information link to Wikipedia. | |
Details | Computed by PubChem 2.1 (PubChem release 2021.05.07) | |
Source | PubChem | |
URL | https://pubchem.ncbi.nlm.nih.gov | |
Description | Data deposited in or computed by PubChem | |
DSSTOX Substance ID |
DTXSID70883589 | |
Record name | Lead(II) oxalate | |
Source | EPA DSSTox | |
URL | https://comptox.epa.gov/dashboard/DTXSID70883589 | |
Description | DSSTox provides a high quality public chemistry resource for supporting improved predictive toxicology. | |
Molecular Weight |
295 g/mol | |
Details | Computed by PubChem 2.1 (PubChem release 2021.08.13) | |
Source | PubChem | |
URL | https://pubchem.ncbi.nlm.nih.gov | |
Description | Data deposited in or computed by PubChem | |
Physical Description |
White solid; [Merck Index] | |
Record name | Lead(II) oxalate | |
Source | Haz-Map, Information on Hazardous Chemicals and Occupational Diseases | |
URL | https://haz-map.com/Agents/2525 | |
Description | Haz-Map® is an occupational health database designed for health and safety professionals and for consumers seeking information about the adverse effects of workplace exposures to chemical and biological agents. | |
Explanation | Copyright (c) 2022 Haz-Map(R). All rights reserved. Unless otherwise indicated, all materials from Haz-Map are copyrighted by Haz-Map(R). No part of these materials, either text or image may be used for any purpose other than for personal use. Therefore, reproduction, modification, storage in a retrieval system or retransmission, in any form or by any means, electronic, mechanical or otherwise, for reasons other than personal use, is strictly prohibited without prior written permission. | |
CAS No. |
814-93-7 | |
Record name | Lead oxalate | |
Source | ChemIDplus | |
URL | https://pubchem.ncbi.nlm.nih.gov/substance/?source=chemidplus&sourceid=0000814937 | |
Description | ChemIDplus is a free, web search system that provides access to the structure and nomenclature authority files used for the identification of chemical substances cited in National Library of Medicine (NLM) databases, including the TOXNET system. | |
Record name | Ethanedioic acid, lead(2+) salt (1:1) | |
Source | EPA Chemicals under the TSCA | |
URL | https://www.epa.gov/chemicals-under-tsca | |
Description | EPA Chemicals under the Toxic Substances Control Act (TSCA) collection contains information on chemicals and their regulations under TSCA, including non-confidential content from the TSCA Chemical Substance Inventory and Chemical Data Reporting. | |
Record name | Lead(II) oxalate | |
Source | EPA DSSTox | |
URL | https://comptox.epa.gov/dashboard/DTXSID70883589 | |
Description | DSSTox provides a high quality public chemistry resource for supporting improved predictive toxicology. | |
Record name | Lead oxalate | |
Source | European Chemicals Agency (ECHA) | |
URL | https://echa.europa.eu/substance-information/-/substanceinfo/100.011.284 | |
Description | The European Chemicals Agency (ECHA) is an agency of the European Union which is the driving force among regulatory authorities in implementing the EU's groundbreaking chemicals legislation for the benefit of human health and the environment as well as for innovation and competitiveness. | |
Explanation | Use of the information, documents and data from the ECHA website is subject to the terms and conditions of this Legal Notice, and subject to other binding limitations provided for under applicable law, the information, documents and data made available on the ECHA website may be reproduced, distributed and/or used, totally or in part, for non-commercial purposes provided that ECHA is acknowledged as the source: "Source: European Chemicals Agency, http://echa.europa.eu/". Such acknowledgement must be included in each copy of the material. ECHA permits and encourages organisations and individuals to create links to the ECHA website under the following cumulative conditions: Links can only be made to webpages that provide a link to the Legal Notice page. | |
Record name | LEAD OXALATE | |
Source | FDA Global Substance Registration System (GSRS) | |
URL | https://gsrs.ncats.nih.gov/ginas/app/beta/substances/642NGP7E5U | |
Description | The FDA Global Substance Registration System (GSRS) enables the efficient and accurate exchange of information on what substances are in regulated products. Instead of relying on names, which vary across regulatory domains, countries, and regions, the GSRS knowledge base makes it possible for substances to be defined by standardized, scientific descriptions. | |
Explanation | Unless otherwise noted, the contents of the FDA website (www.fda.gov), both text and graphics, are not copyrighted. They are in the public domain and may be republished, reprinted and otherwise used freely by anyone without the need to obtain permission from FDA. Credit to the U.S. Food and Drug Administration as the source is appreciated but not required. | |
Retrosynthesis Analysis
AI-Powered Synthesis Planning: Our tool employs the Template_relevance Pistachio, Template_relevance Bkms_metabolic, Template_relevance Pistachio_ringbreaker, Template_relevance Reaxys, Template_relevance Reaxys_biocatalysis model, leveraging a vast database of chemical reactions to predict feasible synthetic routes.
One-Step Synthesis Focus: Specifically designed for one-step synthesis, it provides concise and direct routes for your target compounds, streamlining the synthesis process.
Accurate Predictions: Utilizing the extensive PISTACHIO, BKMS_METABOLIC, PISTACHIO_RINGBREAKER, REAXYS, REAXYS_BIOCATALYSIS database, our tool offers high-accuracy predictions, reflecting the latest in chemical research and data.
Strategy Settings
Precursor scoring | Relevance Heuristic |
---|---|
Min. plausibility | 0.01 |
Model | Template_relevance |
Template Set | Pistachio/Bkms_metabolic/Pistachio_ringbreaker/Reaxys/Reaxys_biocatalysis |
Top-N result to add to graph | 6 |
Feasible Synthetic Routes
Q1: What is the molecular formula and weight of Lead Oxalate?
A1: this compound has the molecular formula PbC2O4 and a molecular weight of 295.21 g/mol. []
Q2: Are there any spectroscopic studies on this compound?
A2: Yes, infrared and Raman spectroscopic studies have been conducted on anhydrous this compound (PbC2O4), providing insights into its structural properties. []
Q3: How does the molecular weight of polyethylene glycol (PEG) affect the morphology of lead oxide produced from this compound?
A3: Research shows that increasing the molecular weight of PEG during this compound synthesis shifts the morphology of the resulting lead oxide from an irregular shape to a rod-like structure. []
Q4: Can this compound be used as a precursor for lead oxide in lead-acid batteries?
A5: Yes, research demonstrates that this compound can be calcined to produce lead oxide (α-PbO and β-PbO) suitable for use as a positive active material in lead-acid batteries. Batteries using this lead oxide exhibited good cyclic stability and high-rate discharge characteristics. []
Q5: What is the effect of the calcination temperature on the lead oxide obtained from this compound?
A6: The calcination temperature influences the ratio of α-PbO and β-PbO in the final product. Lead oxide synthesized at different calcination temperatures comprises varying proportions of α-PbO and β-PbO. []
Q6: Does this compound find application in material synthesis?
A7: Yes, this compound serves as a precursor material in the synthesis of lead zirconate titanate (PZT) powder, a crucial component in electronic ceramics. []
Q7: What is the role of this compound in synthesizing PZT powder?
A8: In a partial this compound coprecipitation method, lead ions react with a (Zr,Ti)O(OH)2 precursor in the presence of oxalic acid. This reaction forms a PZT precursor which, upon calcination, yields well-crystallized perovskite phase PZT powder. []
Q8: Can fungi interact with lead-containing minerals like vanadinite?
A9: Yes, studies show that fungi like Aspergillus niger can bioweather vanadinite [Pb5(VO4)3Cl]. The fungi release oxalic acid, which solubilizes vanadinite, leading to the precipitation of this compound. []
Q9: Do other lead compounds undergo fungal biotransformation?
A10: Yes, fungi can transform various insoluble lead compounds. For instance, Gliocladium roseum has been shown to solubilize lead carbonate (PbCO3), resulting in the precipitation of this compound crystals. [] Similarly, Aspergillus niger can biotransform lead nitrate [Pb(NO3)2] into this compound. []
Q10: Can fungi transform metallic lead?
A11: Research shows that Paecilomyces javanicus can transform metallic lead into different lead compounds. Initially, plumbonacrite [Pb10(CO3)6O(OH)6] forms. After a more extended incubation period, this compound and cerussite (PbCO3) are detected alongside a novel lead hydroxycarbonate. []
Q11: Is there a common mechanism for fungal biotransformation of lead apatites?
A12: Research suggests a general pattern for the biogenic transformation of lead apatite minerals like mimetite [Pb5(AsO4)3Cl], pyromorphite [Pb5(PO4)3Cl], and vanadinite [Pb5(VO4)3Cl] by fungi. Fungi like Aspergillus niger can solubilize these minerals, with the simultaneous precipitation of this compound. []
Q12: How does the presence of oxalate affect the stability of lead vanadate minerals?
A13: Geochemical modeling studies demonstrate that oxalate complexation with vanadium significantly reduces the stability of lead vanadate minerals. This effect promotes the formation of cationic lead(II) and this compound. []
Q13: Can lead be extracted from industrial residues using this compound chemistry?
A14: Research suggests that lead can be leached from sphalerite concentrate direct leaching residue using hydrochloric acid and sodium chloride. The extracted lead, present as [PbCl4]2-, can be precipitated as this compound using sodium oxalate. []
Q14: What analytical techniques are used to characterize this compound and related compounds?
A15: Several analytical techniques are employed, including: * X-ray powder diffraction (XRPD): Used to identify and characterize the crystalline structure of this compound and other lead-containing minerals. [, , , ] * Scanning electron microscopy (SEM): Provides information about the morphology and size of this compound crystals. [, ] * Energy-dispersive X-ray spectroscopy (EDXA): Used in conjunction with SEM to determine the elemental composition of the precipitated minerals, confirming the presence of lead. [] * Fourier transform infrared spectroscopy (FTIR): Helps identify the functional groups present in lead-containing compounds, such as hydroxyl and carbonate groups. [] * Thermogravimetric analysis (TGA): Used to study the thermal behavior and decomposition of this compound and related compounds. [, ]
Q15: Are there environmental concerns regarding this compound?
A16: While this compound can be used in remediation strategies, it's crucial to acknowledge its potential environmental hazards. Lead is a toxic heavy metal, and its release into the environment needs to be carefully managed. []
Q16: What is the significance of responsible lead waste management?
A17: Given the toxicity of lead, proper waste management is crucial. Strategies for resource efficiency and protection, such as recycling, are essential to minimize the environmental impact of lead-containing materials like this compound. []
Q17: Can this compound be formed in industrial settings, posing risks to human health?
A18: Yes, studies highlight the potential for lead exposure and toxicity in industries utilizing lead compounds. For example, paint factory employees exposed to lead naphthenate and this compound exhibited elevated blood lead levels and related health issues. [] This finding underscores the importance of stringent safety measures and regulations in such workplaces.
Disclaimer and Information on In-Vitro Research Products
Please be aware that all articles and product information presented on BenchChem are intended solely for informational purposes. The products available for purchase on BenchChem are specifically designed for in-vitro studies, which are conducted outside of living organisms. In-vitro studies, derived from the Latin term "in glass," involve experiments performed in controlled laboratory settings using cells or tissues. It is important to note that these products are not categorized as medicines or drugs, and they have not received approval from the FDA for the prevention, treatment, or cure of any medical condition, ailment, or disease. We must emphasize that any form of bodily introduction of these products into humans or animals is strictly prohibited by law. It is essential to adhere to these guidelines to ensure compliance with legal and ethical standards in research and experimentation.