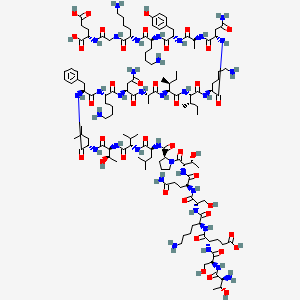
beta-Endorphin (6-31)
Overview
Description
Beta-Endorphin (6-31) is a peptide fragment derived from the larger beta-Endorphin molecule, which is an endogenous opioid peptide. Beta-Endorphin is primarily produced in the pituitary gland and the brain but can also be synthesized and released by leukocytes. It plays significant roles in pain modulation, immune system function, reward, and stress responses .
Mechanism of Action
Target of Action
β-Endorphin (6-31) (human) primarily targets the mu-opioid receptors , which are found throughout the body, brain, and cells of the immune system . These receptors regulate a diverse set of systems . β-Endorphin is a neurohormone secreted by the pituitary gland and reaches all tissues present in the body by diffusion .
Mode of Action
β-Endorphin (6-31) (human) interacts with its targets, the mu-opioid receptors, as an agonist . This interaction results in a variety of physiological effects, including potent analgesic effects . It also plays a role in reward-centric and homeostasis-restoring behaviors .
Biochemical Pathways
β-Endorphin is produced primarily by the anterior lobe of the pituitary gland and in pro-opiomelanocortin (POMC) cells primarily located in the hypothalamus . β-Endorphin and other cleavage products are produced in the multistage processing of POMC primarily involving prohormone convertases (PC) 1 and 2 . This biochemical pathway is crucial for the production and function of β-Endorphin.
Pharmacokinetics
β-Endorphin exhibits a notably high degree of degradation-resistance in the brain . This property, along with its production and release from the pituitary gland, allows it to reach all tissues in the body by diffusion . This widespread distribution contributes to its bioavailability and its ability to exert effects throughout the body.
Result of Action
The interaction of β-Endorphin with mu-opioid receptors leads to a variety of effects. These include potent analgesic effects, modulation of stress response, and involvement in reward-centric and homeostasis-restoring behaviors . It has been associated with hunger, thrill, pain, maternal care, sexual behavior, and reward cognition .
Biochemical Analysis
Biochemical Properties
β-Endorphin (6-31) (human) plays a crucial role in biochemical reactions by acting as an opioid receptor agonist. It preferentially binds to μ-opioid receptors, which are widely distributed in the central and peripheral nervous systems . This interaction leads to the modulation of pain and stress responses. The binding of β-Endorphin (6-31) (human) to μ-opioid receptors triggers a cascade of intracellular events, including the inhibition of adenylate cyclase, reduction of cyclic adenosine monophosphate (cAMP) levels, and activation of potassium channels, resulting in hyperpolarization of neurons and decreased neuronal excitability .
Cellular Effects
β-Endorphin (6-31) (human) exerts various effects on different cell types and cellular processes. In neurons, it modulates neurotransmitter release, thereby influencing pain perception and stress responses . It also affects immune cells by modulating cytokine production and release, which can impact inflammation and immune responses . Additionally, β-Endorphin (6-31) (human) influences cell signaling pathways, gene expression, and cellular metabolism, contributing to its diverse physiological effects .
Molecular Mechanism
The molecular mechanism of β-Endorphin (6-31) (human) involves its binding to μ-opioid receptors, which are G-protein-coupled receptors. Upon binding, β-Endorphin (6-31) (human) induces a conformational change in the receptor, leading to the activation of G-proteins . This activation inhibits adenylate cyclase, reducing cAMP levels and subsequently decreasing protein kinase A (PKA) activity. The reduction in PKA activity leads to the opening of potassium channels and closing of calcium channels, resulting in hyperpolarization and decreased excitability of neurons . Additionally, β-Endorphin (6-31) (human) can modulate gene expression by influencing transcription factors and other regulatory proteins .
Temporal Effects in Laboratory Settings
In laboratory settings, the effects of β-Endorphin (6-31) (human) can vary over time. Studies have shown that β-Endorphin (6-31) (human) is relatively stable and resistant to degradation . Its effects on cellular function can change over time, with prolonged exposure leading to desensitization of opioid receptors and reduced efficacy . Long-term studies have also indicated that β-Endorphin (6-31) (human) can have lasting effects on cellular function, including changes in gene expression and cellular metabolism .
Dosage Effects in Animal Models
The effects of β-Endorphin (6-31) (human) in animal models are dose-dependent. Low doses of β-Endorphin (6-31) (human) have been shown to enhance pain relief and reduce stress responses . Higher doses can lead to adverse effects, including respiratory depression and reduced motor function . Studies have also identified threshold effects, where a certain dosage is required to achieve significant physiological effects .
Metabolic Pathways
β-Endorphin (6-31) (human) is involved in several metabolic pathways, primarily related to its role as an opioid receptor agonist. It interacts with enzymes such as prohormone convertases (PC1 and PC2) and carboxypeptidase E, which are involved in the processing of its precursor, pro-opiomelanocortin (POMC) . These interactions are crucial for the production and regulation of β-Endorphin (6-31) (human) levels in the body .
Transport and Distribution
Within cells and tissues, β-Endorphin (6-31) (human) is transported and distributed through various mechanisms. It binds to transport proteins and receptors that facilitate its movement across cell membranes . In the central nervous system, β-Endorphin (6-31) (human) is distributed through the cerebrospinal fluid, allowing it to reach different regions of the brain and spinal cord . Its distribution is also influenced by its binding to opioid receptors, which are widely distributed in the nervous system .
Subcellular Localization
β-Endorphin (6-31) (human) is localized within specific subcellular compartments, including secretory granules and vesicles in neurons and immune cells . These compartments facilitate the storage and release of β-Endorphin (6-31) (human) in response to physiological stimuli . The subcellular localization of β-Endorphin (6-31) (human) is also influenced by post-translational modifications, such as phosphorylation and glycosylation, which can affect its activity and function .
Preparation Methods
Synthetic Routes and Reaction Conditions
The synthesis of beta-Endorphin (6-31) typically involves solid-phase peptide synthesis (SPPS). This method allows for the sequential addition of amino acids to a growing peptide chain anchored to a solid resin. The process includes:
Coupling Reaction: Each amino acid is activated and coupled to the growing peptide chain.
Deprotection: Removal of protecting groups from the amino acids to allow for the next coupling reaction.
Cleavage: The completed peptide is cleaved from the resin and purified.
Industrial Production Methods
Industrial production of beta-Endorphin (6-31) follows similar principles but on a larger scale. Automated peptide synthesizers are often used to increase efficiency and yield. High-performance liquid chromatography (HPLC) is employed for purification to ensure the peptide’s purity and quality .
Chemical Reactions Analysis
Types of Reactions
Beta-Endorphin (6-31) can undergo various chemical reactions, including:
Oxidation: This reaction can modify the methionine residue in the peptide.
Reduction: Disulfide bonds, if present, can be reduced to free thiols.
Substitution: Amino acid residues can be substituted to create analogs with different properties.
Common Reagents and Conditions
Oxidation: Hydrogen peroxide or other oxidizing agents.
Reduction: Dithiothreitol (DTT) or other reducing agents.
Substitution: Specific amino acid derivatives and coupling reagents like HBTU (O-Benzotriazole-N,N,N’,N’-tetramethyl-uronium-hexafluoro-phosphate).
Major Products
The major products formed from these reactions depend on the specific modifications made. For example, oxidation of methionine results in methionine sulfoxide, while reduction of disulfide bonds yields free thiols .
Scientific Research Applications
Beta-Endorphin (6-31) has a wide range of scientific research applications:
Chemistry: Used as a model peptide for studying peptide synthesis and modification techniques.
Biology: Investigated for its role in cellular signaling and immune responses.
Medicine: Explored for its potential therapeutic effects in pain management, inflammation, and stress-related disorders.
Industry: Utilized in the development of peptide-based drugs and diagnostic tools.
Comparison with Similar Compounds
Similar Compounds
Alpha-Endorphin: Another endogenous opioid peptide with similar but distinct effects.
Gamma-Endorphin: Shares structural similarities with beta-Endorphin but has different receptor affinities and physiological effects.
Enkephalins: Smaller opioid peptides that also bind to opioid receptors but with different potency and duration of action.
Uniqueness of Beta-Endorphin (6-31)
Beta-Endorphin (6-31) is unique due to its specific sequence and the resulting biological activity. It has a higher affinity for certain opioid receptors compared to other endorphins and enkephalins, making it particularly effective in modulating pain and immune responses .
Properties
IUPAC Name |
(2S)-2-[[2-[[(2S)-6-amino-2-[[(2S)-6-amino-2-[[(2S)-2-[[(2S)-2-[[(2S)-4-amino-2-[[(2S)-6-amino-2-[[(2S,3S)-2-[[(2S,3S)-2-[[(2S)-2-[[(2S)-4-amino-2-[[(2S)-6-amino-2-[[(2S)-2-[[(2S)-2-[[(2S,3R)-2-[[(2S)-2-[[(2S)-2-[[(2S)-1-[(2S,3R)-2-[[(2S)-5-amino-2-[[(2S)-2-[[(2S)-6-amino-2-[[(2S)-2-[[(2S)-2-[[(2S,3R)-2-amino-3-hydroxybutanoyl]amino]-3-hydroxypropanoyl]amino]-4-carboxybutanoyl]amino]hexanoyl]amino]-3-hydroxypropanoyl]amino]-5-oxopentanoyl]amino]-3-hydroxybutanoyl]pyrrolidine-2-carbonyl]amino]-4-methylpentanoyl]amino]-3-methylbutanoyl]amino]-3-hydroxybutanoyl]amino]-4-methylpentanoyl]amino]-3-phenylpropanoyl]amino]hexanoyl]amino]-4-oxobutanoyl]amino]propanoyl]amino]-3-methylpentanoyl]amino]-3-methylpentanoyl]amino]hexanoyl]amino]-4-oxobutanoyl]amino]propanoyl]amino]-3-(4-hydroxyphenyl)propanoyl]amino]hexanoyl]amino]hexanoyl]amino]acetyl]amino]pentanedioic acid | |
---|---|---|
Details | Computed by Lexichem TK 2.7.0 (PubChem release 2021.05.07) | |
Source | PubChem | |
URL | https://pubchem.ncbi.nlm.nih.gov | |
Description | Data deposited in or computed by PubChem | |
InChI |
InChI=1S/C131H218N34O40/c1-16-68(9)103(127(200)151-82(38-25-30-54-136)112(185)155-90(60-96(138)173)116(189)142-70(11)107(180)152-88(59-76-40-42-77(171)43-41-76)119(192)147-79(35-22-27-51-133)110(183)145-78(34-21-26-50-132)109(182)141-62-98(175)144-85(131(204)205)46-49-100(178)179)162-128(201)104(69(10)17-2)161-108(181)71(12)143-117(190)91(61-97(139)174)154-111(184)80(36-23-28-52-134)148-120(193)89(58-75-32-19-18-20-33-75)153-118(191)86(56-65(3)4)157-129(202)105(73(14)169)163-126(199)102(67(7)8)160-121(194)87(57-66(5)6)156-124(197)94-39-31-55-165(94)130(203)106(74(15)170)164-115(188)83(44-47-95(137)172)149-122(195)92(63-166)158-113(186)81(37-24-29-53-135)146-114(187)84(45-48-99(176)177)150-123(196)93(64-167)159-125(198)101(140)72(13)168/h18-20,32-33,40-43,65-74,78-94,101-106,166-171H,16-17,21-31,34-39,44-64,132-136,140H2,1-15H3,(H2,137,172)(H2,138,173)(H2,139,174)(H,141,182)(H,142,189)(H,143,190)(H,144,175)(H,145,183)(H,146,187)(H,147,192)(H,148,193)(H,149,195)(H,150,196)(H,151,200)(H,152,180)(H,153,191)(H,154,184)(H,155,185)(H,156,197)(H,157,202)(H,158,186)(H,159,198)(H,160,194)(H,161,181)(H,162,201)(H,163,199)(H,164,188)(H,176,177)(H,178,179)(H,204,205)/t68-,69-,70-,71-,72+,73+,74+,78-,79-,80-,81-,82-,83-,84-,85-,86-,87-,88-,89-,90-,91-,92-,93-,94-,101-,102-,103-,104-,105-,106-/m0/s1 | |
Details | Computed by InChI 1.0.6 (PubChem release 2021.05.07) | |
Source | PubChem | |
URL | https://pubchem.ncbi.nlm.nih.gov | |
Description | Data deposited in or computed by PubChem | |
InChI Key |
WCOFQMXJGOCODK-JXBHHXAKSA-N | |
Details | Computed by InChI 1.0.6 (PubChem release 2021.05.07) | |
Source | PubChem | |
URL | https://pubchem.ncbi.nlm.nih.gov | |
Description | Data deposited in or computed by PubChem | |
Canonical SMILES |
CCC(C)C(C(=O)NC(C(C)CC)C(=O)NC(CCCCN)C(=O)NC(CC(=O)N)C(=O)NC(C)C(=O)NC(CC1=CC=C(C=C1)O)C(=O)NC(CCCCN)C(=O)NC(CCCCN)C(=O)NCC(=O)NC(CCC(=O)O)C(=O)O)NC(=O)C(C)NC(=O)C(CC(=O)N)NC(=O)C(CCCCN)NC(=O)C(CC2=CC=CC=C2)NC(=O)C(CC(C)C)NC(=O)C(C(C)O)NC(=O)C(C(C)C)NC(=O)C(CC(C)C)NC(=O)C3CCCN3C(=O)C(C(C)O)NC(=O)C(CCC(=O)N)NC(=O)C(CO)NC(=O)C(CCCCN)NC(=O)C(CCC(=O)O)NC(=O)C(CO)NC(=O)C(C(C)O)N | |
Details | Computed by OEChem 2.3.0 (PubChem release 2021.05.07) | |
Source | PubChem | |
URL | https://pubchem.ncbi.nlm.nih.gov | |
Description | Data deposited in or computed by PubChem | |
Isomeric SMILES |
CC[C@H](C)[C@@H](C(=O)N[C@@H]([C@@H](C)CC)C(=O)N[C@@H](CCCCN)C(=O)N[C@@H](CC(=O)N)C(=O)N[C@@H](C)C(=O)N[C@@H](CC1=CC=C(C=C1)O)C(=O)N[C@@H](CCCCN)C(=O)N[C@@H](CCCCN)C(=O)NCC(=O)N[C@@H](CCC(=O)O)C(=O)O)NC(=O)[C@H](C)NC(=O)[C@H](CC(=O)N)NC(=O)[C@H](CCCCN)NC(=O)[C@H](CC2=CC=CC=C2)NC(=O)[C@H](CC(C)C)NC(=O)[C@H]([C@@H](C)O)NC(=O)[C@H](C(C)C)NC(=O)[C@H](CC(C)C)NC(=O)[C@@H]3CCCN3C(=O)[C@H]([C@@H](C)O)NC(=O)[C@H](CCC(=O)N)NC(=O)[C@H](CO)NC(=O)[C@H](CCCCN)NC(=O)[C@H](CCC(=O)O)NC(=O)[C@H](CO)NC(=O)[C@H]([C@@H](C)O)N | |
Details | Computed by OEChem 2.3.0 (PubChem release 2021.05.07) | |
Source | PubChem | |
URL | https://pubchem.ncbi.nlm.nih.gov | |
Description | Data deposited in or computed by PubChem | |
Molecular Formula |
C131H218N34O40 | |
Details | Computed by PubChem 2.1 (PubChem release 2021.05.07) | |
Source | PubChem | |
URL | https://pubchem.ncbi.nlm.nih.gov | |
Description | Data deposited in or computed by PubChem | |
Molecular Weight |
2909.3 g/mol | |
Details | Computed by PubChem 2.1 (PubChem release 2021.05.07) | |
Source | PubChem | |
URL | https://pubchem.ncbi.nlm.nih.gov | |
Description | Data deposited in or computed by PubChem | |
Disclaimer and Information on In-Vitro Research Products
Please be aware that all articles and product information presented on BenchChem are intended solely for informational purposes. The products available for purchase on BenchChem are specifically designed for in-vitro studies, which are conducted outside of living organisms. In-vitro studies, derived from the Latin term "in glass," involve experiments performed in controlled laboratory settings using cells or tissues. It is important to note that these products are not categorized as medicines or drugs, and they have not received approval from the FDA for the prevention, treatment, or cure of any medical condition, ailment, or disease. We must emphasize that any form of bodily introduction of these products into humans or animals is strictly prohibited by law. It is essential to adhere to these guidelines to ensure compliance with legal and ethical standards in research and experimentation.