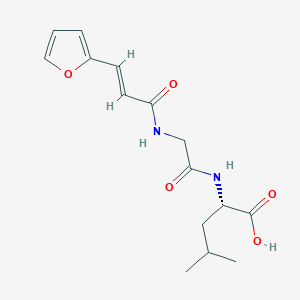
FA-Gly-leu-OH
Overview
Description
Mechanism of Action
Target of Action
FA-Gly-Leu-OH, also known as Larazotide , primarily targets the tight junctions in the intestinal epithelium . These junctions play a crucial role in maintaining the barrier function of the intestines, preventing the passage of harmful substances from the gut into the bloodstream .
Mode of Action
Larazotide functions as a tight junction regulator . It reverses leaky junctions to their normally closed state by blocking zonulin receptors . Zonulin is a protein that modulates the permeability of tight junctions between cells in the wall of the digestive tract .
Biochemical Pathways
In conditions like celiac disease, fragments of gliadin protein can pass the intestinal epithelium and trigger an immune response . This process begins with the binding of indigestible gliadin fragments to the chemokine CXC motif receptor 3 (CXCR3) on the luminal side of the intestinal epithelium . This leads to the induction of myeloid differentiation factor 88 (MYD88) and the release of zonulin into the lumen . Zonulin then binds to epidermal growth factor receptor (EGFR) and protease-activated receptor 2 (PAR2) in the intestinal epithelium . This complex initiates a signaling pathway that results in tight junction disassembly and increased intestinal permeability . Larazotide intervenes in this pathway by blocking zonulin receptors, thereby preventing tight junction disassembly and the associated increase in intestinal permeability .
Pharmacokinetics
It’s known that the use of biocompatible and biodegradable polymers for drug conjugation, like in the case of larazotide, can enhance the clinical efficacy of drugs . They can improve the drug’s aqueous solubility, bioavailability, and prolong its circulation time in the blood .
Result of Action
The primary result of Larazotide’s action is the reduction of intestinal permeability . By preventing the disassembly of tight junctions, it helps maintain the barrier function of the intestines, reducing the passage of harmful substances from the gut into the bloodstream .
Action Environment
The safety data sheet for this compound suggests that it should be stored at temperatures below -15°C . This implies that the stability of the compound could be influenced by temperature.
Biochemical Analysis
Biochemical Properties
FA-Gly-leu-OH interacts with several enzymes, particularly proteases, which are capable of cleaving it at the Gly-Leu peptide bond . The cleavage of the tripeptide is more efficient and leads to a decrease in absorbance at 340 nm, which can be monitored continuously using spectrophotometric assays .
Cellular Effects
For instance, DT-109 has been reported to reduce steatohepatitis and hepatic fibrosis in non-human primates .
Molecular Mechanism
The molecular mechanism of this compound primarily involves its interaction with proteases. The cleavage of this compound by these enzymes is considered the rate-limiting step in certain biochemical reactions . The efficiency of this cleavage can be influenced by factors such as temperature .
Temporal Effects in Laboratory Settings
The temporal effects of this compound in laboratory settings are largely dependent on the specific assays in which it is used. For instance, in spectrophotometric assays, the rate of decrease in absorbance at 340 nm following the cleavage of this compound can be monitored continuously .
Dosage Effects in Animal Models
For example, DT-109 (Gly-Gly-Leu) has been reported to dose-dependently attenuate steatohepatitis and fibrosis in mice .
Preparation Methods
Synthetic Routes and Reaction Conditions
The synthesis of FA-Gly-leu-OH typically involves solid-phase peptide synthesis (SPPS), a widely used method for creating peptides. The process begins with the attachment of the first amino acid, N-formylglycine, to a solid resin. Subsequent amino acids, glycine and leucine, are added sequentially through coupling reactions facilitated by reagents such as N,N’-diisopropylcarbodiimide (DIC) and hydroxybenzotriazole (HOBt) . The peptide chain is then cleaved from the resin using trifluoroacetic acid (TFA), yielding the final product.
Industrial Production Methods
In an industrial setting, the synthesis of this compound can be scaled up using automated peptide synthesizers. These machines streamline the SPPS process, allowing for the efficient production of large quantities of the peptide. The use of environmentally friendly solvents, such as water, is also being explored to reduce the environmental impact of peptide synthesis .
Chemical Reactions Analysis
Types of Reactions
FA-Gly-leu-OH undergoes various chemical reactions, including hydrolysis, transpeptidation, and oxidation .
Common Reagents and Conditions
Hydrolysis: This reaction involves the cleavage of the peptide bond in the presence of water and enzymes such as peptidases.
Transpeptidation: This reaction involves the transfer of a terminal amino acid from one peptide chain to another, facilitated by enzymes.
Oxidation: This reaction can occur under oxidative conditions, leading to the formation of disulfide bonds or other oxidized products.
Major Products Formed
The major products formed from these reactions include shorter peptide fragments, modified peptides, and oxidized derivatives .
Scientific Research Applications
FA-Gly-leu-OH has several scientific research applications:
Biochemistry: It is used as a substrate for studying enzyme activity, particularly peptidases.
Pharmacology: It serves as a model compound for developing peptide-based drugs and studying their pharmacokinetics and pharmacodynamics.
Industrial Chemistry: It is utilized in the synthesis of peptide-based materials and as a building block for more complex peptides.
Comparison with Similar Compounds
Similar Compounds
Gly-leu-OH: This compound lacks the N-formyl group present in FA-Gly-leu-OH, making it less reactive in certain enzymatic reactions.
FA-Gly-Phe-OH: This compound contains phenylalanine instead of leucine, which alters its interaction with enzymes and its overall reactivity.
Uniqueness
This compound is unique due to the presence of the N-formyl group, which enhances its stability and reactivity in enzymatic reactions. This makes it a valuable tool for studying enzyme mechanisms and developing peptide-based therapeutics.
Properties
IUPAC Name |
(2S)-2-[[2-[[(E)-3-(furan-2-yl)prop-2-enoyl]amino]acetyl]amino]-4-methylpentanoic acid | |
---|---|---|
Details | Computed by LexiChem 2.6.6 (PubChem release 2019.06.18) | |
Source | PubChem | |
URL | https://pubchem.ncbi.nlm.nih.gov | |
Description | Data deposited in or computed by PubChem | |
InChI |
InChI=1S/C15H20N2O5/c1-10(2)8-12(15(20)21)17-14(19)9-16-13(18)6-5-11-4-3-7-22-11/h3-7,10,12H,8-9H2,1-2H3,(H,16,18)(H,17,19)(H,20,21)/b6-5+/t12-/m0/s1 | |
Details | Computed by InChI 1.0.5 (PubChem release 2019.06.18) | |
Source | PubChem | |
URL | https://pubchem.ncbi.nlm.nih.gov | |
Description | Data deposited in or computed by PubChem | |
InChI Key |
OBFWNISOMLCYSX-FYJFLYSWSA-N | |
Details | Computed by InChI 1.0.5 (PubChem release 2019.06.18) | |
Source | PubChem | |
URL | https://pubchem.ncbi.nlm.nih.gov | |
Description | Data deposited in or computed by PubChem | |
Canonical SMILES |
CC(C)CC(C(=O)O)NC(=O)CNC(=O)C=CC1=CC=CO1 | |
Details | Computed by OEChem 2.1.5 (PubChem release 2019.06.18) | |
Source | PubChem | |
URL | https://pubchem.ncbi.nlm.nih.gov | |
Description | Data deposited in or computed by PubChem | |
Isomeric SMILES |
CC(C)C[C@@H](C(=O)O)NC(=O)CNC(=O)/C=C/C1=CC=CO1 | |
Details | Computed by OEChem 2.1.5 (PubChem release 2019.06.18) | |
Source | PubChem | |
URL | https://pubchem.ncbi.nlm.nih.gov | |
Description | Data deposited in or computed by PubChem | |
Molecular Formula |
C15H20N2O5 | |
Details | Computed by PubChem 2.1 (PubChem release 2019.06.18) | |
Source | PubChem | |
URL | https://pubchem.ncbi.nlm.nih.gov | |
Description | Data deposited in or computed by PubChem | |
Molecular Weight |
308.33 g/mol | |
Details | Computed by PubChem 2.1 (PubChem release 2021.05.07) | |
Source | PubChem | |
URL | https://pubchem.ncbi.nlm.nih.gov | |
Description | Data deposited in or computed by PubChem | |
Retrosynthesis Analysis
AI-Powered Synthesis Planning: Our tool employs the Template_relevance Pistachio, Template_relevance Bkms_metabolic, Template_relevance Pistachio_ringbreaker, Template_relevance Reaxys, Template_relevance Reaxys_biocatalysis model, leveraging a vast database of chemical reactions to predict feasible synthetic routes.
One-Step Synthesis Focus: Specifically designed for one-step synthesis, it provides concise and direct routes for your target compounds, streamlining the synthesis process.
Accurate Predictions: Utilizing the extensive PISTACHIO, BKMS_METABOLIC, PISTACHIO_RINGBREAKER, REAXYS, REAXYS_BIOCATALYSIS database, our tool offers high-accuracy predictions, reflecting the latest in chemical research and data.
Strategy Settings
Precursor scoring | Relevance Heuristic |
---|---|
Min. plausibility | 0.01 |
Model | Template_relevance |
Template Set | Pistachio/Bkms_metabolic/Pistachio_ringbreaker/Reaxys/Reaxys_biocatalysis |
Top-N result to add to graph | 6 |
Feasible Synthetic Routes
Disclaimer and Information on In-Vitro Research Products
Please be aware that all articles and product information presented on BenchChem are intended solely for informational purposes. The products available for purchase on BenchChem are specifically designed for in-vitro studies, which are conducted outside of living organisms. In-vitro studies, derived from the Latin term "in glass," involve experiments performed in controlled laboratory settings using cells or tissues. It is important to note that these products are not categorized as medicines or drugs, and they have not received approval from the FDA for the prevention, treatment, or cure of any medical condition, ailment, or disease. We must emphasize that any form of bodily introduction of these products into humans or animals is strictly prohibited by law. It is essential to adhere to these guidelines to ensure compliance with legal and ethical standards in research and experimentation.