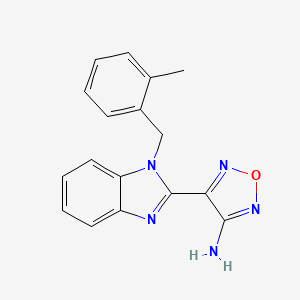
3'-O-Methyluridine
Overview
Description
3’-O-Methyluridine is a modified nucleoside with the molecular formula C10H14N2O6. It is a derivative of uridine, where the hydroxyl group at the 3’ position of the ribose sugar is replaced by a methoxy group.
Mechanism of Action
Target of Action
3’-O-Methyluridine, also known as N3-methyluridine, is a pyrimidine nucleoside . It primarily targets ribosomal RNAs (rRNAs) in living organisms, where it is present as an RNA modification . This modification has been detected in 23S rRNA of archaea, 16S and 23S rRNA of eubacteria, and 18S, 25S, and 28S of eukaryotic ribosomal RNAs . The role of rRNA is crucial in protein synthesis, where it provides a mechanism for decoding mRNA into amino acids and interacts with the tRNAs during translation .
Mode of Action
This modification likely influences the structure and function of the rRNA, potentially affecting the process of protein synthesis .
Biochemical Pathways
The biochemical pathways affected by 3’-O-Methyluridine involve the formation of modified ribonucleosides in RNA. The evolutionarily conserved proteins MnmE and GidA are known to catalyze the formation of two methyluridine derivatives at tRNA wobble positions . These modifications play a critical role in decoding NNG/A codons and maintaining the reading frame during mRNA translation .
Pharmacokinetics
Studies on similar compounds, such as [5‐3h]‐2′‐o‐methyluridine, suggest that these compounds are rapidly distributed throughout the body following intravenous administration . Three metabolites, namely uridine (M1), cytidine (M2), and uracil (M3), were identified as the major circulating components, representing 32.8%, 8.11%, and 23.6% of radioactivity area under the curve, respectively . Renal excretion accounted for about 52.7% of the dose .
Result of Action
The molecular and cellular effects of 3’-O-Methyluridine’s action are primarily related to its role in modifying rRNA. These modifications can influence the process of protein synthesis, potentially affecting the structure and function of proteins within the cell .
Biochemical Analysis
Biochemical Properties
3’-O-Methyluridine is involved in the methylation of 2′ and 3′ hydroxyl groups of uridines in plant microRNAs . This biochemical reaction involves enzymes and proteins that interact with 3’-O-Methyluridine, leading to the formation of methylated uridines .
Cellular Effects
It is known that uridine, a similar compound, plays a pivotal role in various cellular processes, including macromolecule synthesis, circadian rhythms, inflammatory response, antioxidant process, and aging . It is reasonable to speculate that 3’-O-Methyluridine may have similar effects on cells.
Molecular Mechanism
It is known that uridine, a similar compound, is critical to glycogen synthesis through the formation of uridine diphosphate glucose (UDPG), which promotes the production of UDP-GlcNAc in the hexosamine biosynthetic pathway and supplies UDP-GlcNAc for O-GlcNAcylation . This process can regulate protein modification and affect its function .
Temporal Effects in Laboratory Settings
It is known that uridine, a similar compound, has long-term effects on cellular function .
Dosage Effects in Animal Models
It is known that uridine, a similar compound, has dose-dependent effects on apoptotic markers in animal models .
Metabolic Pathways
3’-O-Methyluridine is involved in the methylation of 2′ and 3′ hydroxyl groups of uridines in plant microRNAs . This process is part of the larger metabolic pathway involving the formation of methylated uridines .
Transport and Distribution
It is known that uridine, a similar compound, enters cells through nucleoside transporter .
Subcellular Localization
It is known that uridine, a similar compound, is found in plasma and cerebrospinal fluid with a concentration higher than the other nucleosides .
Preparation Methods
Synthetic Routes and Reaction Conditions: The synthesis of 3’-O-Methyluridine typically involves the methylation of uridine. One common method is the use of methyl iodide in the presence of a base such as sodium hydride or potassium carbonate. The reaction is carried out in an aprotic solvent like dimethylformamide or dimethyl sulfoxide at elevated temperatures .
Industrial Production Methods: Industrial production of 3’-O-Methyluridine may involve similar methylation reactions but on a larger scale. The process is optimized for higher yields and purity, often using continuous flow reactors and advanced purification techniques such as high-performance liquid chromatography .
Chemical Reactions Analysis
Types of Reactions: 3’-O-Methyluridine can undergo various chemical reactions, including:
Oxidation: It can be oxidized to form uridine derivatives with altered biological activities.
Reduction: Reduction reactions can modify the uracil base or the ribose sugar.
Substitution: The methoxy group can be substituted with other functional groups under specific conditions.
Common Reagents and Conditions:
Oxidation: Common oxidizing agents include potassium permanganate and hydrogen peroxide.
Reduction: Reducing agents such as sodium borohydride or lithium aluminum hydride are used.
Substitution: Nucleophilic substitution reactions may involve reagents like sodium azide or thiols.
Major Products: The major products formed from these reactions depend on the specific conditions and reagents used. For example, oxidation may yield uridine-5’-aldehyde, while substitution reactions can produce various 3’-substituted uridine derivatives .
Scientific Research Applications
3’-O-Methyluridine has several applications in scientific research:
Chemistry: It is used in studies of nucleoside analogs and their chemical properties.
Biology: It plays a role in the study of RNA methylation and its effects on gene expression.
Medicine: Modified nucleosides like 3’-O-Methyluridine are investigated for their potential as antiviral and anticancer agents.
Industry: It is used in the synthesis of nucleic acid-based drugs and as a biochemical probe in various assays
Comparison with Similar Compounds
2’-O-Methyluridine: Another methylated uridine derivative with the methoxy group at the 2’ position.
5-Methyluridine: A derivative with a methyl group at the 5 position of the uracil base.
2’-O-Methylcytidine: A methylated cytidine analog with the methoxy group at the 2’ position
Uniqueness: 3’-O-Methyluridine is unique due to its specific modification at the 3’ position, which can lead to distinct biological effects compared to other methylated nucleosides.
Properties
IUPAC Name |
1-[(2R,3R,4S,5R)-3-hydroxy-5-(hydroxymethyl)-4-methoxyoxolan-2-yl]pyrimidine-2,4-dione | |
---|---|---|
Details | Computed by Lexichem TK 2.7.0 (PubChem release 2021.05.07) | |
Source | PubChem | |
URL | https://pubchem.ncbi.nlm.nih.gov | |
Description | Data deposited in or computed by PubChem | |
InChI |
InChI=1S/C10H14N2O6/c1-17-8-5(4-13)18-9(7(8)15)12-3-2-6(14)11-10(12)16/h2-3,5,7-9,13,15H,4H2,1H3,(H,11,14,16)/t5-,7-,8-,9-/m1/s1 | |
Details | Computed by InChI 1.0.6 (PubChem release 2021.05.07) | |
Source | PubChem | |
URL | https://pubchem.ncbi.nlm.nih.gov | |
Description | Data deposited in or computed by PubChem | |
InChI Key |
YKNATSNMFLEFRB-ZOQUXTDFSA-N | |
Details | Computed by InChI 1.0.6 (PubChem release 2021.05.07) | |
Source | PubChem | |
URL | https://pubchem.ncbi.nlm.nih.gov | |
Description | Data deposited in or computed by PubChem | |
Canonical SMILES |
COC1C(OC(C1O)N2C=CC(=O)NC2=O)CO | |
Details | Computed by OEChem 2.3.0 (PubChem release 2021.05.07) | |
Source | PubChem | |
URL | https://pubchem.ncbi.nlm.nih.gov | |
Description | Data deposited in or computed by PubChem | |
Isomeric SMILES |
CO[C@@H]1[C@H](O[C@H]([C@@H]1O)N2C=CC(=O)NC2=O)CO | |
Details | Computed by OEChem 2.3.0 (PubChem release 2021.05.07) | |
Source | PubChem | |
URL | https://pubchem.ncbi.nlm.nih.gov | |
Description | Data deposited in or computed by PubChem | |
Molecular Formula |
C10H14N2O6 | |
Details | Computed by PubChem 2.1 (PubChem release 2021.05.07) | |
Source | PubChem | |
URL | https://pubchem.ncbi.nlm.nih.gov | |
Description | Data deposited in or computed by PubChem | |
DSSTOX Substance ID |
DTXSID501346818 | |
Record name | 3'-O-Methyluridine | |
Source | EPA DSSTox | |
URL | https://comptox.epa.gov/dashboard/DTXSID501346818 | |
Description | DSSTox provides a high quality public chemistry resource for supporting improved predictive toxicology. | |
Molecular Weight |
258.23 g/mol | |
Details | Computed by PubChem 2.1 (PubChem release 2021.05.07) | |
Source | PubChem | |
URL | https://pubchem.ncbi.nlm.nih.gov | |
Description | Data deposited in or computed by PubChem | |
CAS No. |
6038-59-1 | |
Record name | 3'-O-Methyluridine | |
Source | EPA DSSTox | |
URL | https://comptox.epa.gov/dashboard/DTXSID501346818 | |
Description | DSSTox provides a high quality public chemistry resource for supporting improved predictive toxicology. | |
Retrosynthesis Analysis
AI-Powered Synthesis Planning: Our tool employs the Template_relevance Pistachio, Template_relevance Bkms_metabolic, Template_relevance Pistachio_ringbreaker, Template_relevance Reaxys, Template_relevance Reaxys_biocatalysis model, leveraging a vast database of chemical reactions to predict feasible synthetic routes.
One-Step Synthesis Focus: Specifically designed for one-step synthesis, it provides concise and direct routes for your target compounds, streamlining the synthesis process.
Accurate Predictions: Utilizing the extensive PISTACHIO, BKMS_METABOLIC, PISTACHIO_RINGBREAKER, REAXYS, REAXYS_BIOCATALYSIS database, our tool offers high-accuracy predictions, reflecting the latest in chemical research and data.
Strategy Settings
Precursor scoring | Relevance Heuristic |
---|---|
Min. plausibility | 0.01 |
Model | Template_relevance |
Template Set | Pistachio/Bkms_metabolic/Pistachio_ringbreaker/Reaxys/Reaxys_biocatalysis |
Top-N result to add to graph | 6 |
Feasible Synthetic Routes
Disclaimer and Information on In-Vitro Research Products
Please be aware that all articles and product information presented on BenchChem are intended solely for informational purposes. The products available for purchase on BenchChem are specifically designed for in-vitro studies, which are conducted outside of living organisms. In-vitro studies, derived from the Latin term "in glass," involve experiments performed in controlled laboratory settings using cells or tissues. It is important to note that these products are not categorized as medicines or drugs, and they have not received approval from the FDA for the prevention, treatment, or cure of any medical condition, ailment, or disease. We must emphasize that any form of bodily introduction of these products into humans or animals is strictly prohibited by law. It is essential to adhere to these guidelines to ensure compliance with legal and ethical standards in research and experimentation.