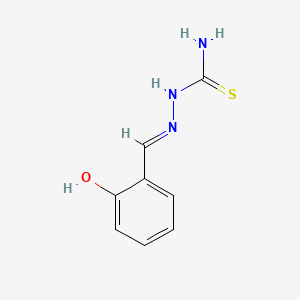
Salicylaldehyde thiosemicarbazone
Overview
Description
Salicylaldehyde thiosemicarbazone is a chemical compound derived from the condensation reaction between salicylaldehyde and thiosemicarbazide. It is known for its diverse applications in various fields, including chemistry, biology, medicine, and industry. The compound exhibits significant biological activities, making it a subject of interest in scientific research.
Mechanism of Action
Target of Action
Salicylaldehyde thiosemicarbazone (STSC) and its derivatives have been reported to form high stability complexes with transition metal ions in solution . These complexes are known to interact with various targets, including the influenza virus PA endonuclease , a critical enzyme in the viral replication process . The presence of the OH group in STSC derivatives might provide a more favorable coordination for hard metal ions like Mg2+ that prefers oxygen donor atoms .
Mode of Action
The mode of action of STSC involves the formation of complexes with metal ions, which can then interact with their targets . For instance, in the case of influenza virus PA endonuclease, it is assumed that STSC inhibitors chelate the divalent metal ions (Mg2+ or Mn2+) in the enzyme’s catalytic site . The probable mechanistic action involves modification in lipophilicity regulating the entry into the cell, which is altered upon coordination of metal ions .
Biochemical Pathways
STSC affects various biochemical pathways. Initial studies have shown that thiosemicarbazone can inhibit ribonucleotide reductase activity and further impede DNA synthesis, finally exerting its anti-cancer effect . The mechanism of action involves most probably the formation of an iron(II)–Triapine complex, which reacts with molecular oxygen to result in the generation of reactive oxygen species (ROS) .
Pharmacokinetics
The pharmacokinetic properties of STSC and its copper (II) complexes can be tuned by e.g., the attachment of electron-donating substituents or via various conjugations . The novel conjugates are more lipophilic and possess higher membrane permeability than STSC based on the n-octanol/water partitioning and the parallel artificial membrane permeability assays .
Result of Action
The result of STSC’s action can be seen in its cytotoxic effects against several human cancer cell lines . The novel ligands thn-TSC and estrone–TSC were found to be only moderately cytotoxic against several human cancer cell lines; however rather low IC50 values were measured in the hormone-responsive MCF-7 breast cancer cell lines .
Action Environment
The action of STSC is influenced by various environmental factors. The complexes are fairly stable at pH 7.4, and are able to oxidize glutathione readily . The observed stability order is STSC < thn-TSC < estrone–TSC . The novel ligands thn-TSC and estrone–TSC were found to be only moderately cytotoxic against several human cancer cell lines .
Biochemical Analysis
Biochemical Properties
Salicylaldehyde thiosemicarbazone plays a crucial role in biochemical reactions by interacting with various enzymes, proteins, and other biomolecules. One of the notable interactions is with the influenza virus PA endonuclease, where this compound acts as an inhibitor . This interaction involves the chelation of divalent metal ions such as magnesium or manganese at the enzyme’s catalytic site, thereby inhibiting its activity. Additionally, this compound has shown interactions with proteins involved in cancer cell proliferation, such as quinone reductase-2, indicating its potential as an anticancer agent .
Cellular Effects
This compound exhibits significant effects on various types of cells and cellular processes. In cancer cells, it has been observed to induce cytotoxicity, leading to cell death. For instance, studies have shown that this compound derivatives exhibit potent cytotoxic activity against C6 glioma and MCF7 breast cancer cell lines . This compound influences cell function by affecting cell signaling pathways, gene expression, and cellular metabolism. It has been reported to interfere with the replication of the influenza virus in MDCK cells by inhibiting the PA endonuclease .
Molecular Mechanism
The molecular mechanism of action of this compound involves several key processes. At the molecular level, this compound exerts its effects through binding interactions with biomolecules. For example, it chelates metal ions in the catalytic site of the influenza virus PA endonuclease, thereby inhibiting the enzyme’s activity . Additionally, this compound has been shown to inhibit quinone reductase-2, which plays a role in cancer cell proliferation . These interactions result in the inhibition of enzyme activity and subsequent changes in gene expression.
Temporal Effects in Laboratory Settings
In laboratory settings, the effects of this compound have been observed to change over time. The stability and degradation of this compound are critical factors that influence its long-term effects on cellular function. Studies have shown that this compound maintains its stability under specific conditions, allowing for sustained biological activity . Prolonged exposure to certain environmental factors may lead to its degradation, potentially reducing its efficacy. Long-term studies in vitro and in vivo have demonstrated that this compound can have lasting effects on cellular function, including sustained inhibition of viral replication and cancer cell proliferation .
Dosage Effects in Animal Models
The effects of this compound vary with different dosages in animal models. Studies have indicated that there is a threshold effect, where low doses of this compound may not exhibit significant biological activity, while higher doses result in pronounced effects . At optimal dosages, this compound has shown potent antiviral and anticancer activities. At excessively high doses, toxic or adverse effects may be observed, highlighting the importance of dosage optimization in therapeutic applications .
Metabolic Pathways
This compound is involved in various metabolic pathways, interacting with enzymes and cofactors that influence its biological activity. For instance, it has been shown to interact with quinone reductase-2, an enzyme involved in cellular redox regulation . This interaction affects the metabolic flux and levels of metabolites within the cell. Additionally, this compound’s chelation of metal ions in the catalytic site of the influenza virus PA endonuclease impacts the enzyme’s activity and subsequent viral replication .
Transport and Distribution
The transport and distribution of this compound within cells and tissues are critical for its biological activity. This compound is transported across cell membranes and distributed to various cellular compartments. It interacts with transporters and binding proteins that facilitate its localization and accumulation within specific tissues . The distribution of this compound influences its efficacy in targeting viral replication and cancer cell proliferation.
Subcellular Localization
This compound exhibits specific subcellular localization, which affects its activity and function. This compound is directed to particular compartments or organelles within the cell, where it exerts its biological effects. Targeting signals and post-translational modifications play a role in directing this compound to its site of action . For example, its localization to the nucleus may be essential for inhibiting the influenza virus PA endonuclease and preventing viral replication .
Preparation Methods
Synthetic Routes and Reaction Conditions: Salicylaldehyde thiosemicarbazone is typically synthesized through the condensation reaction of salicylaldehyde with thiosemicarbazide. The reaction is carried out in an ethanol solution, often under reflux conditions to ensure complete reaction. The product is then purified through recrystallization from ethanol .
Industrial Production Methods: While specific industrial production methods for this compound are not extensively documented, the synthesis generally follows the laboratory preparation methods, scaled up to meet industrial demands. The use of optimized reaction conditions, such as microwave-assisted synthesis, can enhance yield and efficiency .
Chemical Reactions Analysis
Types of Reactions: Salicylaldehyde thiosemicarbazone undergoes various chemical reactions, including:
Oxidation: The compound can be oxidized to form corresponding sulfoxides or sulfones.
Reduction: Reduction reactions can convert the thiosemicarbazone moiety to thiosemicarbazide.
Substitution: The compound can participate in substitution reactions, where the thiosemicarbazone group is replaced by other functional groups.
Common Reagents and Conditions:
Oxidation: Common oxidizing agents include hydrogen peroxide and potassium permanganate.
Reduction: Reducing agents such as sodium borohydride or lithium aluminum hydride are used.
Substitution: Various electrophiles and nucleophiles can be employed under appropriate conditions.
Major Products Formed:
Oxidation: Sulfoxides and sulfones.
Reduction: Thiosemicarbazide derivatives.
Substitution: Functionalized thiosemicarbazone derivatives.
Scientific Research Applications
Salicylaldehyde thiosemicarbazone has a wide range of applications in scientific research:
Comparison with Similar Compounds
- Estrone–salicylaldehyde thiosemicarbazone hybrid (estrone–TSC)
- 5,6,7,8-tetrahydro-1-naphtol thiosemicarbazone (thn-TSC)
- 2-hydroxyacetophenone thiosemicarbazone
- 2-hydroxynaphthaldehyde thiosemicarbazone
Comparison: this compound is unique due to its specific structure, which allows it to form stable metal complexes and exhibit significant biological activities. Compared to similar compounds, it has higher membrane permeability and lipophilicity, enhancing its potential as a therapeutic agent . Additionally, its ability to generate ROS and induce oxidative stress distinguishes it from other thiosemicarbazone derivatives .
Properties
IUPAC Name |
[(E)-(2-hydroxyphenyl)methylideneamino]thiourea | |
---|---|---|
Details | Computed by Lexichem TK 2.7.0 (PubChem release 2021.05.07) | |
Source | PubChem | |
URL | https://pubchem.ncbi.nlm.nih.gov | |
Description | Data deposited in or computed by PubChem | |
InChI |
InChI=1S/C8H9N3OS/c9-8(13)11-10-5-6-3-1-2-4-7(6)12/h1-5,12H,(H3,9,11,13)/b10-5+ | |
Details | Computed by InChI 1.0.6 (PubChem release 2021.05.07) | |
Source | PubChem | |
URL | https://pubchem.ncbi.nlm.nih.gov | |
Description | Data deposited in or computed by PubChem | |
InChI Key |
OHNSRYBTSVDDKA-BJMVGYQFSA-N | |
Details | Computed by InChI 1.0.6 (PubChem release 2021.05.07) | |
Source | PubChem | |
URL | https://pubchem.ncbi.nlm.nih.gov | |
Description | Data deposited in or computed by PubChem | |
Canonical SMILES |
C1=CC=C(C(=C1)C=NNC(=S)N)O | |
Details | Computed by OEChem 2.3.0 (PubChem release 2021.05.07) | |
Source | PubChem | |
URL | https://pubchem.ncbi.nlm.nih.gov | |
Description | Data deposited in or computed by PubChem | |
Isomeric SMILES |
C1=CC=C(C(=C1)/C=N/NC(=S)N)O | |
Details | Computed by OEChem 2.3.0 (PubChem release 2021.05.07) | |
Source | PubChem | |
URL | https://pubchem.ncbi.nlm.nih.gov | |
Description | Data deposited in or computed by PubChem | |
Molecular Formula |
C8H9N3OS | |
Details | Computed by PubChem 2.1 (PubChem release 2021.05.07) | |
Source | PubChem | |
URL | https://pubchem.ncbi.nlm.nih.gov | |
Description | Data deposited in or computed by PubChem | |
Molecular Weight |
195.24 g/mol | |
Details | Computed by PubChem 2.1 (PubChem release 2021.05.07) | |
Source | PubChem | |
URL | https://pubchem.ncbi.nlm.nih.gov | |
Description | Data deposited in or computed by PubChem | |
Solubility |
26 [ug/mL] (The mean of the results at pH 7.4) | |
Record name | SID26671022 | |
Source | Burnham Center for Chemical Genomics | |
URL | https://pubchem.ncbi.nlm.nih.gov/bioassay/1996#section=Data-Table | |
Description | Aqueous solubility in buffer at pH 7.4 | |
CAS No. |
5351-90-6 | |
Record name | Salicylaldehyde thiosemicarbazone | |
Source | ChemIDplus | |
URL | https://pubchem.ncbi.nlm.nih.gov/substance/?source=chemidplus&sourceid=0005351906 | |
Description | ChemIDplus is a free, web search system that provides access to the structure and nomenclature authority files used for the identification of chemical substances cited in National Library of Medicine (NLM) databases, including the TOXNET system. | |
Record name | Salicylaldehyde thiosemicarbazone | |
Source | DTP/NCI | |
URL | https://dtp.cancer.gov/dtpstandard/servlet/dwindex?searchtype=NSC&outputformat=html&searchlist=201642 | |
Description | The NCI Development Therapeutics Program (DTP) provides services and resources to the academic and private-sector research communities worldwide to facilitate the discovery and development of new cancer therapeutic agents. | |
Explanation | Unless otherwise indicated, all text within NCI products is free of copyright and may be reused without our permission. Credit the National Cancer Institute as the source. | |
Record name | Salicylaldehyde thiosemicarbazone | |
Source | DTP/NCI | |
URL | https://dtp.cancer.gov/dtpstandard/servlet/dwindex?searchtype=NSC&outputformat=html&searchlist=733 | |
Description | The NCI Development Therapeutics Program (DTP) provides services and resources to the academic and private-sector research communities worldwide to facilitate the discovery and development of new cancer therapeutic agents. | |
Explanation | Unless otherwise indicated, all text within NCI products is free of copyright and may be reused without our permission. Credit the National Cancer Institute as the source. | |
Retrosynthesis Analysis
AI-Powered Synthesis Planning: Our tool employs the Template_relevance Pistachio, Template_relevance Bkms_metabolic, Template_relevance Pistachio_ringbreaker, Template_relevance Reaxys, Template_relevance Reaxys_biocatalysis model, leveraging a vast database of chemical reactions to predict feasible synthetic routes.
One-Step Synthesis Focus: Specifically designed for one-step synthesis, it provides concise and direct routes for your target compounds, streamlining the synthesis process.
Accurate Predictions: Utilizing the extensive PISTACHIO, BKMS_METABOLIC, PISTACHIO_RINGBREAKER, REAXYS, REAXYS_BIOCATALYSIS database, our tool offers high-accuracy predictions, reflecting the latest in chemical research and data.
Strategy Settings
Precursor scoring | Relevance Heuristic |
---|---|
Min. plausibility | 0.01 |
Model | Template_relevance |
Template Set | Pistachio/Bkms_metabolic/Pistachio_ringbreaker/Reaxys/Reaxys_biocatalysis |
Top-N result to add to graph | 6 |
Feasible Synthetic Routes
Q1: What is Salicylaldehyde thiosemicarbazone (STSC) and what makes it interesting for scientific research?
A: this compound (STSC) is an organic compound belonging to the thiosemicarbazone family. It's known for its ability to form complexes with various metal ions, a property that underpins its diverse biological and chemical applications. [, , , , , ]
Q2: How does STSC interact with metal ions, and what are the structural implications?
A: STSC typically acts as a tridentate ligand, meaning it can bind to a metal ion through three donor atoms: the phenolic oxygen, the azomethine nitrogen, and the thione/thiol sulfur. This binding forms stable five- and six-membered chelate rings with the metal ion. The exact coordination mode can vary depending on the metal ion, substituents on the STSC molecule, and the reaction conditions. [, , , , ]
Q3: What is the significance of the light-induced excited-spin-state trapping (LIESST) phenomenon observed in some Iron(III) STSC complexes?
A: Research indicates that incorporating electron-withdrawing groups like -Cl or -Br at the 5-position of the salicylaldehyde moiety in Iron(III) STSC complexes can induce the LIESST effect. This phenomenon, characterized by the trapping of the excited high-spin state upon light irradiation, has implications for developing molecular switches and data storage devices. Interestingly, the halogen-free STSC complex does not exhibit this behavior, highlighting the crucial role of substituents in dictating the compound's photophysical properties. []
Q4: What spectroscopic techniques are commonly used to characterize STSC and its metal complexes?
A: Researchers utilize a range of spectroscopic techniques to elucidate the structural and electronic characteristics of STSC and its metal complexes. These techniques include: * Infrared (IR) spectroscopy: Provides information about the functional groups present in the molecule, particularly the characteristic stretching frequencies of C=N, C=S, and O-H bonds. [, , , , , , ] * Nuclear Magnetic Resonance (NMR) spectroscopy: Offers detailed insights into the structure and dynamics of the molecule by analyzing the magnetic properties of atomic nuclei. Both 1H and 13C NMR, along with multinuclear NMR for specific applications, are commonly employed. [, , , , , , ] * UV-Vis spectroscopy: Helps study the electronic transitions within the molecule, providing information about the ligand and metal ion interactions and the complex's geometry. [, , , , , ] * Electron Paramagnetic Resonance (EPR) spectroscopy: Useful for characterizing paramagnetic metal complexes, offering insights into the metal ion's electronic configuration and its coordination environment. [, ] * Mass spectrometry: Helps determine the molecular weight and fragmentation pattern of the compound, providing structural information. [, , , ] * X-ray diffraction: For solid-state analysis, this technique reveals the crystal structure and molecular packing of STSC and its complexes. [, , , , , , ]
Q5: How do different solvents influence the structural conformation of STSC derivatives?
A: Studies utilizing NMR and DFT calculations reveal that the choice of solvent significantly impacts the molecular conformation and hydrogen bonding patterns in STSC derivatives. Solvents with varying polarities, and therefore differing proton donor and acceptor abilities, can influence the equilibrium between various tautomeric forms of STSC, ultimately affecting its structure and potentially its biological activity. [, ]
Q6: How does STSC behave as a semiconductor, and what influences its conductivity?
A: Research on the electrical conductivity of STSC and its palladium(II), copper(II), and ruthenium(III) complexes suggests that these compounds exhibit semiconductive behavior. The study reveals that the temperature significantly influences the conductivity, and the activation energies calculated for these compounds provide valuable insights into the conduction mechanism. []
Q7: Can you elaborate on the application of STSC in the development of chemically modified electrodes?
A: STSC demonstrates its versatility in analytical chemistry by serving as a modifying agent for electrodes. A study highlights the use of a chemically modified electrode (CME) containing STSC for preconcentrating copper(II) ions before their quantification via voltammetry. This method displays high sensitivity and selectivity for copper(II) detection, showcasing the potential of STSC-based CMEs in analytical applications. []
Q8: What is the role of STSC in the development of ion-selective electrodes?
A: STSC has proven to be a valuable ionophore in developing ion-selective electrodes. One study successfully employed STSC to create a PVC membrane-based tin-selective electrode. This electrode exhibited a Nernstian response to Sn2+, demonstrating the potential of STSC as a sensing element for specific metal ions. [, ]
Q9: How do copper(II) and cadmium(II) complexes of STSC compare in terms of their radical scavenging activity?
A: Research indicates a significant difference in the radical scavenging activity between copper(II) and cadmium(II) complexes of STSC. The copper(II) complex exhibits considerably higher activity compared to the free ligand, Cu2+ ions, and the cadmium(II) complex. This enhanced activity is attributed to a synergistic effect between the ligand structure and the copper ion's ability to switch between different oxidation states, facilitating electron transfer during the radical scavenging process. []
Q10: What is the significance of the substituent effect on the biological activity of STSC derivatives?
A: Studies on fluoreneazo-salicylaldehyde thiosemicarbazone derivatives, synthesized by reacting fluorene, salicylaldehyde, and thiosemicarbazone, reveal a clear relationship between the nature of the substituent group and the compound's biological activity, particularly its plant growth-regulating properties. This finding underscores the importance of considering substituent effects when designing STSC derivatives for specific biological applications. []
Q11: How does computational chemistry contribute to understanding STSC and its derivatives?
A: Computational chemistry plays a vital role in predicting and explaining the properties of STSC and its derivatives. Density functional theory (DFT) calculations, in particular, provide valuable insights into the electronic structure, geometry, and reactivity of these compounds. These calculations help establish a structure-activity relationship, facilitating the design of new STSC derivatives with tailored properties. [, , ]
Q12: What are the potential applications of STSC and its derivatives based on the available research?
A: The diverse properties of STSC and its metal complexes render them promising candidates for a wide range of applications, including: * Pharmaceuticals: STSC derivatives exhibit a broad spectrum of biological activities, including antibacterial, antifungal, antiviral, antimalarial, and antitumor properties. This makes them attractive targets for developing novel therapeutic agents. [, , , , ] * Sensors: Their ability to selectively bind to specific metal ions makes them suitable for developing electrochemical sensors and optical probes for various analytes. [, ] * Materials science: The semiconducting properties of STSC and its complexes open possibilities for applications in electronic devices. [] * Catalysis: Metal complexes of STSC can act as catalysts in various organic reactions, offering potential applications in synthetic chemistry. []
Disclaimer and Information on In-Vitro Research Products
Please be aware that all articles and product information presented on BenchChem are intended solely for informational purposes. The products available for purchase on BenchChem are specifically designed for in-vitro studies, which are conducted outside of living organisms. In-vitro studies, derived from the Latin term "in glass," involve experiments performed in controlled laboratory settings using cells or tissues. It is important to note that these products are not categorized as medicines or drugs, and they have not received approval from the FDA for the prevention, treatment, or cure of any medical condition, ailment, or disease. We must emphasize that any form of bodily introduction of these products into humans or animals is strictly prohibited by law. It is essential to adhere to these guidelines to ensure compliance with legal and ethical standards in research and experimentation.