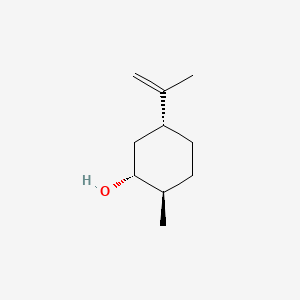
(-)-Dihydrocarveol
Overview
Description
(-)-Dihydrocarveol is a monoterpenoid alcohol belonging to the p-menthane family, with the molecular formula C₁₀H₁₈O and a molecular weight of 154.25 g/mol . It features three chiral centers, leading to eight possible stereoisomers, with the (1R,2R,5R)-configuration being the most studied . Naturally occurring in plants such as Michelia alba (44–65% in bud stages) and Mentha species, it contributes to green, spearmint, and peppery odor profiles in essential oils . Industrially, it is synthesized via catalytic hydrogenation of carvone or through biocatalytic methods using ene-reductases and ketoreductases, achieving high stereoselectivity (40–93% yield, 91–99% enantiomeric excess) . Applications span fragrances, cosmetics, and household products due to its versatile aroma .
Preparation Methods
Synthetic Routes and Reaction Conditions:
Hydrogenation of Carvone: One of the primary methods to synthesize (-)-dihydrocarveol is through the hydrogenation of carvone. This reaction typically involves the use of a hydrogenation catalyst such as palladium on carbon (Pd/C) under mild conditions. The reaction proceeds as follows: [ \text{Carvone} + \text{H}_2 \rightarrow \text{this compound} ]
Reduction of Carvone: Another method involves the reduction of carvone using sodium borohydride (NaBH4) or lithium aluminum hydride (LiAlH4) as reducing agents. This method is often preferred for laboratory-scale synthesis due to its simplicity and high yield.
Industrial Production Methods: In industrial settings, the hydrogenation of carvone is the preferred method due to its scalability and cost-effectiveness. The process involves the use of large-scale hydrogenation reactors and efficient catalysts to ensure high yield and purity of this compound.
Chemical Reactions Analysis
Types of Reactions:
Oxidation: (-)-Dihydrocarveol can undergo oxidation reactions to form carvone or other oxidized derivatives. Common oxidizing agents include potassium permanganate (KMnO4) and chromium trioxide (CrO3).
Reduction: The compound can be further reduced to form dihydrocarveyl acetate using acetic anhydride and a catalyst.
Substitution: this compound can participate in substitution reactions where the hydroxyl group is replaced by other functional groups. For example, reacting with thionyl chloride (SOCl2) can replace the hydroxyl group with a chlorine atom.
Common Reagents and Conditions:
Oxidation: Potassium permanganate (KMnO4), chromium trioxide (CrO3)
Reduction: Sodium borohydride (NaBH4), lithium aluminum hydride (LiAlH4)
Substitution: Thionyl chloride (SOCl2), acetic anhydride
Major Products:
Oxidation: Carvone, other oxidized derivatives
Reduction: Dihydrocarveyl acetate
Substitution: Chlorinated derivatives
Scientific Research Applications
Chemical Properties and Safety Profile
(-)-Dihydrocarveol (CAS # 619-01-2) is characterized as a p-menthane monoterpenoid. It exhibits a range of biological activities and has been evaluated for safety in various contexts. Notably, studies have shown that it does not present significant genotoxic risks, with no mutagenic activity observed in bacterial assays (Ames test) . Moreover, it has been assessed for skin sensitization and reproductive toxicity, indicating a favorable safety profile for its use in consumer products .
Fragrance Industry
One of the primary applications of this compound is in the fragrance industry. It is used as a component in perfumes and personal care products due to its pleasant scent profile. The compound's safety assessment indicates that it can be used without significant risk to consumers .
Table 1: Fragrance Applications of this compound
Application Type | Description |
---|---|
Perfumes | Used as a fragrance component |
Personal Care Products | Incorporated into lotions and creams |
Household Products | Found in air fresheners and cleaning agents |
Agricultural Applications
This compound has shown potential as an acaricide , which is crucial for pest control in agricultural settings. Its efficacy against certain pests makes it a candidate for organic farming practices where synthetic pesticides are avoided.
Case Study: Efficacy as an Acaricide
Research indicates that this compound effectively reduces pest populations while being less harmful to beneficial insects compared to traditional chemical pesticides . This dual action supports sustainable agricultural practices.
Microbial Metabolism
Recent studies have highlighted the role of this compound in microbial metabolism. For instance, Rhodococcus erythropolis can utilize this compound as a sole carbon source, demonstrating its potential in bioremediation processes . The metabolic pathways involved include oxidation to produce carvone and other intermediates, which can be further utilized by the microorganism.
Table 2: Microbial Metabolism of this compound
Microorganism | Metabolic Pathway Description |
---|---|
Rhodococcus erythropolis | Degradation via oxidation to carvone |
Enzymatic Activities | Dihydrocarveol dehydrogenase, monooxygenases |
Synthesis and Research Applications
In the field of synthetic organic chemistry, this compound serves as an important building block for synthesizing various natural products and flavoring agents. Its stereochemistry allows for diverse synthetic pathways, making it valuable for researchers looking to develop new compounds with specific properties .
Table 3: Research Applications
Research Area | Application |
---|---|
Organic Synthesis | Building block for terpenoid synthesis |
Flavor Industry | Additive in food flavoring |
Biochemical Studies | Investigating terpenoid reactions |
Mechanism of Action
The mechanism of action of (-)-dihydrocarveol involves its interaction with cellular membranes and enzymes. It is believed to exert its antimicrobial effects by disrupting the cell membrane integrity of microorganisms, leading to cell lysis and death. The anti-inflammatory effects are thought to be mediated through the inhibition of pro-inflammatory cytokines and enzymes such as cyclooxygenase (COX).
Comparison with Similar Compounds
Structural and Functional Analogues
Key structurally related compounds include carveol, carvone, dihydrocarvone, and neodihydrocarveol. These share the p-menthane skeleton but differ in functional groups and oxidation states (Table 1).
Table 1: Structural Comparison of (-)-Dihydrocarveol and Analogues
Compound | Functional Group | Oxidation State | Key Stereoisomers | Odor Profile |
---|---|---|---|---|
This compound | Alcohol (-OH) | Reduced | 8 stereoisomers | Green, spearmint, pepper |
Carveol | Alcohol (-OH) | Partially oxidized | 4 diastereomers | Minty, herbal |
Carvone | Ketone (=O) | Oxidized | 2 enantiomers | Spearmint, caraway |
Dihydrocarvone | Ketone (=O) | Reduced | 4 stereoisomers | Minty, less pungent |
Neodihydrocarveol | Alcohol (-OH) | Reduced | 1 stereoisomer | Similar to dihydrocarveol |
Occurrence in Natural Sources
This compound and its analogues are distributed across plant taxa but vary in abundance (Table 2).
Table 2: Occurrence in Essential Oils
Plant Species | Compound | Percentage (%) | Developmental Stage/Role |
---|---|---|---|
Michelia alba | Dihydrocarveol | 44–65 | Bud stages (S5–S8) |
Mentha acuminata | Dihydrocarveol | 23.6 | Mature leaves |
Mentha armillaris | Dihydrocarveol | 9.0 | Aerial parts |
Chinese Mentha spp. | Dihydrocarvone | 0.8–15.7 | Aerial parts |
Mentha styphelioides | Methyl eugenol | 91.1 | Leaves (dominant over terpenoids) |
Key Findings :
- Dihydrocarveol dominates in Michelia alba during early bud development, while linalool (floral) prevails in later stages .
- Carvone is more abundant in Mentha species (up to 65.4%), suggesting species-specific chemotypes .
Odor Profiles :
- This compound: Green, spearmint, and pepper notes, critical in perfumes and detergents .
- Carvone : Strong spearmint aroma, used in chewing gums and oral care products .
- Linalool: Floral, dominant in mature Michelia alba flowers, contrasting dihydrocarveol’s green notes .
Stereochemical Considerations
This compound’s eight stereoisomers exhibit subtle odor differences. For example:
- (1R,2S,4S)-Dihydrocarveol : 25.3% yield via Lactobacillus spp. .
- (1S,2S,4S)-Dihydrocarveol : 63% yield in the same system, highlighting microbial stereoselectivity .
In contrast, carvone has only two enantiomers (R and S), with R-carvone being spearmint-like and S-carvone resembling caraway .
Biological Activity
(-)-Dihydrocarveol is a monoterpenoid alcohol derived from the essential oil of various plants, particularly those in the mint family. Its biological activities have garnered attention in recent years, particularly for its antimicrobial, antioxidant, and potential therapeutic effects. This article provides a detailed overview of the biological activity of this compound, supported by case studies and research findings.
Chemical Structure and Properties
This compound has the molecular formula C10H18O and is characterized by a bicyclic structure with hydroxyl and isopropenyl groups. This unique structure contributes to its diverse biological activities.
Antimicrobial Activity
Research has demonstrated that this compound exhibits significant antimicrobial properties against various pathogens. A study indicated that it effectively inhibited the growth of Fusarium sporotrichioides , a filamentous fungus responsible for corn contamination. The compound altered the morphology of fungal hyphae, leading to increased membrane permeability and cytoplasmic loss at elevated concentrations .
Table 1: Antimicrobial Efficacy of this compound
Pathogen | Minimum Inhibitory Concentration (MIC) |
---|---|
Fusarium sporotrichioides | 6.44% |
Klebsiella spp. | 0.6 mg/mL |
Pseudomonas spp. | 1.2 mg/mL |
Antioxidant Activity
This compound has been evaluated for its antioxidant potential using various assays. In particular, studies have shown that it possesses moderate antioxidant activity with an IC50 value indicating its efficacy in scavenging free radicals . This property is crucial for protecting cells from oxidative stress, which is implicated in numerous diseases.
Table 2: Antioxidant Activity of this compound
Method | IC50 Value (µg/mL) |
---|---|
DPPH | 775.3 ± 1.3 |
ABTS | 963.3 ± 1.6 |
Metabolic Pathways
The metabolism of this compound has been studied in microorganisms such as Rhodococcus erythropolis. This bacterium can utilize this compound as a sole carbon source, demonstrating its potential for bioremediation applications. The metabolic pathway involves several enzymatic reactions leading to the formation of intermediate compounds like carvone and dihydrocarvone .
Case Studies
- Antifungal Activity : A study focusing on the antifungal properties of essential oils containing this compound showed promising results against Fusarium species, indicating its potential use as a natural antifungal agent in agriculture .
- Antioxidant Effects : In vivo studies involving animal models have shown that exposure to this compound can enhance antioxidant enzyme activities, suggesting its role in mitigating oxidative stress-related conditions .
Q & A
Basic Research Questions
Q. What are the primary synthetic routes for (-)-Dihydrocarveol, and how do they address stereochemical control?
this compound is synthesized via enzymatic catalysis, particularly using ketoreductases (KREDs) and ene-reductases (EREDs). For example, asymmetric reduction of (R)- or (S)-carvone with KREDs yields carveol intermediates, which are further hydrogenated to dihydrocarveol. Anti-Prelog or Prelog stereopreference in KREDs enables access to specific stereoisomers with diastereomeric excess (de) up to 95% . Key steps include:
- Substrate preparation (e.g., carvone isomer selection).
- Enzyme screening for stereoselectivity.
- Reaction optimization (pH, cofactors like NADPH).
- Post-reaction purification (e.g., column chromatography).
Enzyme Type | Substrate | Product Stereoisomer | Diastereomeric Excess (de) |
---|---|---|---|
KRED (Prelog) | (S)-Carvone | (1S,5R)-Dihydrocarveol | >99% |
ERED | (R)-Carvone | (1R,5S)-Dihydrocarvone | 85% |
Q. Which analytical methods are critical for verifying the stereochemical purity of this compound?
Chiral chromatography (e.g., HPLC with chiral columns) and nuclear magnetic resonance (NMR) with Mosher’s ester derivatization are standard. For example, modified Mosher’s method assigns absolute configurations by comparing -NMR shifts of diastereomeric esters . GC-MS is used for quantifying dihydrocarveol in natural extracts, requiring calibration with authentic standards .
Q. How is this compound identified and quantified in natural essential oils?
Gas chromatography-mass spectrometry (GC-MS) coupled with retention index matching is standard. For instance, in C. officinalis essential oil, dihydrocarveol is identified at 6.9% abundance via GC-MS peaks aligned with reference libraries. Researchers must validate findings with multiple columns (e.g., polar vs. nonpolar) to resolve co-eluting terpenes .
Advanced Research Questions
Q. How can enzymatic synthesis of this compound be optimized for industrial scalability without commercial solvents?
- Substrate engineering : Use carvone dissolved in biocompatible solvents (e.g., cyclopentyl methyl ether) to enhance enzyme stability.
- Cofactor recycling : NADPH regeneration systems (e.g., glucose dehydrogenase) reduce costs .
- Immobilized enzymes : Encapsulate KREDs/EREDs in silica gels or polymers for reusability.
- One-pot cascades : Combine ERED-mediated dihydrocarvone synthesis with KRED reduction to minimize intermediate isolation .
Q. What experimental strategies resolve contradictions in reported dihydrocarveol content across essential oil studies?
Discrepancies (e.g., 6.9% in Egyptian oils vs. trace amounts in others) arise from plant genotype, extraction methods, or analytical variability. Mitigation strategies:
- Standardized protocols : Use hydrodistillation at controlled temperatures (100–120°C) to avoid thermal degradation.
- Interlaboratory validation : Share samples between labs to calibrate GC-MS settings.
- Metabolomic profiling : Compare terpene synthase activity in plant sources to explain compositional differences .
Q. How can computational modeling improve the enantioselectivity of ketoreductases in dihydrocarveol synthesis?
Molecular docking (e.g., AutoDock Vina) predicts enzyme-substrate interactions to guide mutagenesis. For example, mutating KRED active-site residues (e.g., Tyr152→Ala) may alter hydrogen-bonding networks, favoring anti-Prelog selectivity. MD simulations assess conformational stability of enzyme-substrate complexes .
Q. What are the challenges in isolating this compound stereoisomers, and how are they addressed?
Challenges include low natural abundance and similar physicochemical properties of stereoisomers. Solutions:
- Dynamic kinetic resolution : Use lipases to selectively esterify one enantiomer.
- Crystallization-induced diastereomer resolution : Form diastereomeric salts with chiral acids (e.g., tartaric acid).
- Preparative SFC : Supercritical fluid chromatography with chiral stationary phases achieves baseline separation .
Q. Methodological Best Practices
- Data presentation : Use tables to compare enzymatic yields (e.g., de%, reaction time) and figures for chromatographic traces. Label axes with SI units and error bars for triplicate runs .
- Statistical analysis : Apply ANOVA to assess significance of variables (e.g., pH, temperature) on reaction outcomes. Report p-values and confidence intervals .
- Ethical considerations : Disclose conflicts of interest if using proprietary enzymes or datasets .
Properties
IUPAC Name |
(1R,2R,5R)-2-methyl-5-prop-1-en-2-ylcyclohexan-1-ol | |
---|---|---|
Source | PubChem | |
URL | https://pubchem.ncbi.nlm.nih.gov | |
Description | Data deposited in or computed by PubChem | |
InChI |
InChI=1S/C10H18O/c1-7(2)9-5-4-8(3)10(11)6-9/h8-11H,1,4-6H2,2-3H3/t8-,9-,10-/m1/s1 | |
Source | PubChem | |
URL | https://pubchem.ncbi.nlm.nih.gov | |
Description | Data deposited in or computed by PubChem | |
InChI Key |
KRCZYMFUWVJCLI-OPRDCNLKSA-N | |
Source | PubChem | |
URL | https://pubchem.ncbi.nlm.nih.gov | |
Description | Data deposited in or computed by PubChem | |
Canonical SMILES |
CC1CCC(CC1O)C(=C)C | |
Source | PubChem | |
URL | https://pubchem.ncbi.nlm.nih.gov | |
Description | Data deposited in or computed by PubChem | |
Isomeric SMILES |
C[C@@H]1CC[C@H](C[C@H]1O)C(=C)C | |
Source | PubChem | |
URL | https://pubchem.ncbi.nlm.nih.gov | |
Description | Data deposited in or computed by PubChem | |
Molecular Formula |
C10H18O | |
Source | PubChem | |
URL | https://pubchem.ncbi.nlm.nih.gov | |
Description | Data deposited in or computed by PubChem | |
DSSTOX Substance ID |
DTXSID70885700 | |
Record name | Cyclohexanol, 2-methyl-5-(1-methylethenyl)-, (1R,2R,5R)-rel- | |
Source | EPA DSSTox | |
URL | https://comptox.epa.gov/dashboard/DTXSID70885700 | |
Description | DSSTox provides a high quality public chemistry resource for supporting improved predictive toxicology. | |
Molecular Weight |
154.25 g/mol | |
Source | PubChem | |
URL | https://pubchem.ncbi.nlm.nih.gov | |
Description | Data deposited in or computed by PubChem | |
CAS No. |
38049-26-2, 20549-47-7 | |
Record name | Dihydrocarveol | |
Source | CAS Common Chemistry | |
URL | https://commonchemistry.cas.org/detail?cas_rn=38049-26-2 | |
Description | CAS Common Chemistry is an open community resource for accessing chemical information. Nearly 500,000 chemical substances from CAS REGISTRY cover areas of community interest, including common and frequently regulated chemicals, and those relevant to high school and undergraduate chemistry classes. This chemical information, curated by our expert scientists, is provided in alignment with our mission as a division of the American Chemical Society. | |
Explanation | The data from CAS Common Chemistry is provided under a CC-BY-NC 4.0 license, unless otherwise stated. | |
Record name | (1R,2R,5R)-2-Methyl-5-(1-methylethenyl)cyclohexanol | |
Source | CAS Common Chemistry | |
URL | https://commonchemistry.cas.org/detail?cas_rn=20549-47-7 | |
Description | CAS Common Chemistry is an open community resource for accessing chemical information. Nearly 500,000 chemical substances from CAS REGISTRY cover areas of community interest, including common and frequently regulated chemicals, and those relevant to high school and undergraduate chemistry classes. This chemical information, curated by our expert scientists, is provided in alignment with our mission as a division of the American Chemical Society. | |
Explanation | The data from CAS Common Chemistry is provided under a CC-BY-NC 4.0 license, unless otherwise stated. | |
Record name | Dihydrocarveol, (-)- | |
Source | ChemIDplus | |
URL | https://pubchem.ncbi.nlm.nih.gov/substance/?source=chemidplus&sourceid=0020549477 | |
Description | ChemIDplus is a free, web search system that provides access to the structure and nomenclature authority files used for the identification of chemical substances cited in National Library of Medicine (NLM) databases, including the TOXNET system. | |
Record name | Cyclohexanol, 2-methyl-5-(1-methylethenyl)-, (1R,2R,5R)-rel- | |
Source | EPA Chemicals under the TSCA | |
URL | https://www.epa.gov/chemicals-under-tsca | |
Description | EPA Chemicals under the Toxic Substances Control Act (TSCA) collection contains information on chemicals and their regulations under TSCA, including non-confidential content from the TSCA Chemical Substance Inventory and Chemical Data Reporting. | |
Record name | Cyclohexanol, 2-methyl-5-(1-methylethenyl)-, (1R,2R,5R)-rel- | |
Source | EPA DSSTox | |
URL | https://comptox.epa.gov/dashboard/DTXSID70885700 | |
Description | DSSTox provides a high quality public chemistry resource for supporting improved predictive toxicology. | |
Record name | (1α,2β,5α)-2-methyl-5-(1-methylvinyl)cyclohexan-1-ol | |
Source | European Chemicals Agency (ECHA) | |
URL | https://echa.europa.eu/substance-information/-/substanceinfo/100.048.851 | |
Description | The European Chemicals Agency (ECHA) is an agency of the European Union which is the driving force among regulatory authorities in implementing the EU's groundbreaking chemicals legislation for the benefit of human health and the environment as well as for innovation and competitiveness. | |
Explanation | Use of the information, documents and data from the ECHA website is subject to the terms and conditions of this Legal Notice, and subject to other binding limitations provided for under applicable law, the information, documents and data made available on the ECHA website may be reproduced, distributed and/or used, totally or in part, for non-commercial purposes provided that ECHA is acknowledged as the source: "Source: European Chemicals Agency, http://echa.europa.eu/". Such acknowledgement must be included in each copy of the material. ECHA permits and encourages organisations and individuals to create links to the ECHA website under the following cumulative conditions: Links can only be made to webpages that provide a link to the Legal Notice page. | |
Record name | DIHYDROCARVEOL, (-)- | |
Source | FDA Global Substance Registration System (GSRS) | |
URL | https://gsrs.ncats.nih.gov/ginas/app/beta/substances/2683FD21WA | |
Description | The FDA Global Substance Registration System (GSRS) enables the efficient and accurate exchange of information on what substances are in regulated products. Instead of relying on names, which vary across regulatory domains, countries, and regions, the GSRS knowledge base makes it possible for substances to be defined by standardized, scientific descriptions. | |
Explanation | Unless otherwise noted, the contents of the FDA website (www.fda.gov), both text and graphics, are not copyrighted. They are in the public domain and may be republished, reprinted and otherwise used freely by anyone without the need to obtain permission from FDA. Credit to the U.S. Food and Drug Administration as the source is appreciated but not required. | |
Retrosynthesis Analysis
AI-Powered Synthesis Planning: Our tool employs the Template_relevance Pistachio, Template_relevance Bkms_metabolic, Template_relevance Pistachio_ringbreaker, Template_relevance Reaxys, Template_relevance Reaxys_biocatalysis model, leveraging a vast database of chemical reactions to predict feasible synthetic routes.
One-Step Synthesis Focus: Specifically designed for one-step synthesis, it provides concise and direct routes for your target compounds, streamlining the synthesis process.
Accurate Predictions: Utilizing the extensive PISTACHIO, BKMS_METABOLIC, PISTACHIO_RINGBREAKER, REAXYS, REAXYS_BIOCATALYSIS database, our tool offers high-accuracy predictions, reflecting the latest in chemical research and data.
Strategy Settings
Precursor scoring | Relevance Heuristic |
---|---|
Min. plausibility | 0.01 |
Model | Template_relevance |
Template Set | Pistachio/Bkms_metabolic/Pistachio_ringbreaker/Reaxys/Reaxys_biocatalysis |
Top-N result to add to graph | 6 |
Feasible Synthetic Routes
Disclaimer and Information on In-Vitro Research Products
Please be aware that all articles and product information presented on BenchChem are intended solely for informational purposes. The products available for purchase on BenchChem are specifically designed for in-vitro studies, which are conducted outside of living organisms. In-vitro studies, derived from the Latin term "in glass," involve experiments performed in controlled laboratory settings using cells or tissues. It is important to note that these products are not categorized as medicines or drugs, and they have not received approval from the FDA for the prevention, treatment, or cure of any medical condition, ailment, or disease. We must emphasize that any form of bodily introduction of these products into humans or animals is strictly prohibited by law. It is essential to adhere to these guidelines to ensure compliance with legal and ethical standards in research and experimentation.