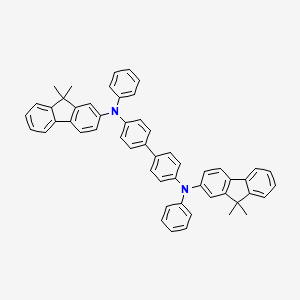
N,N'-Bis(9,9-dimethyl-9H-fluoren-2-yl)-N,N'-diphenylbenzidine
Overview
Description
N,N’-Bis(9,9-dimethyl-9H-fluoren-2-yl)-N,N’-diphenylbenzidine is a complex organic compound known for its unique structural properties and applications in various scientific fields. This compound is particularly notable for its use in organic electronics, such as organic light-emitting diodes (OLEDs) and organic solar cells, due to its excellent hole-transporting capabilities .
Mechanism of Action
Target of Action
BF-DPB primarily targets organic light-emitting diodes (OLEDs) . It serves as an efficient hole transport material (HTL) in these devices . The role of HTLs is to facilitate the movement of positive charges (holes) within the device, which is crucial for the functioning of OLEDs .
Mode of Action
BF-DPB interacts with its targets by enabling more efficient hole injection from itself to the adjacent electron blocking layer . This is attributed to the high ionization potential of BF-DPB . The interaction results in a significantly lower driving voltage, leading to improved performance of the OLEDs .
Biochemical Pathways
The compound’s high ionization potential allows for efficient hole injection, which is a critical step in the operation of OLEDs .
Pharmacokinetics
In the context of oleds, the compound’s properties allow for efficient energy transfer and low operating voltage .
Result of Action
The action of BF-DPB results in OLEDs achieving high luminous efficacy and brightness at a very low voltage . Specifically, BF-DPB based OLEDs can reach a brightness of 10,000 cd/m² at a very low voltage of 3.2 V .
Biochemical Analysis
Biochemical Properties
BF-DPB plays a significant role in biochemical reactions, particularly in the context of organic light-emitting diodes (OLEDs) and other electronic applications. It interacts with various enzymes, proteins, and other biomolecules, influencing their activity and stability. For instance, BF-DPB has been shown to interact with carboxylesterase enzymes, which are involved in the hydrolysis of ester bonds . These interactions are primarily mediated through van der Waals forces and hydrogen bonding, which stabilize the enzyme-substrate complex and enhance the catalytic efficiency of the enzyme.
Cellular Effects
BF-DPB exerts notable effects on various types of cells and cellular processes. It has been observed to influence cell function by modulating cell signaling pathways, gene expression, and cellular metabolism. In particular, BF-DPB affects the balance between cell division and endoreplication in plant cells by interacting with transcription factors such as E2FC and DPB . This interaction regulates the expression of genes involved in the cell cycle, leading to changes in cell proliferation and differentiation.
Molecular Mechanism
The molecular mechanism of BF-DPB involves its ability to modulate charge-transfer states at donor-acceptor heterojunctions. BF-DPB acts as a donor molecule, facilitating the separation of charge-transfer states into free charge carriers . This process is influenced by the molecular packing and orientation of BF-DPB, which enhances its ability to interact with acceptor molecules and promote efficient charge separation. Additionally, BF-DPB has been shown to interact with various biomolecules through non-covalent interactions, such as hydrogen bonding and van der Waals forces, which contribute to its overall biochemical activity .
Temporal Effects in Laboratory Settings
In laboratory settings, the effects of BF-DPB have been studied over time to understand its stability, degradation, and long-term impact on cellular function. BF-DPB exhibits high stability under various conditions, maintaining its structural integrity and biochemical activity over extended periods . Prolonged exposure to certain environmental factors, such as light and temperature, can lead to gradual degradation and reduced efficacy. Long-term studies have shown that BF-DPB can influence cellular function by modulating gene expression and metabolic pathways, leading to sustained changes in cellular behavior .
Dosage Effects in Animal Models
The effects of BF-DPB vary with different dosages in animal models. At low doses, BF-DPB has been shown to enhance cellular function and promote metabolic activity without causing significant adverse effects . At higher doses, BF-DPB can induce toxic effects, including oxidative stress and cellular damage. These threshold effects highlight the importance of optimizing the dosage of BF-DPB to achieve the desired biochemical outcomes while minimizing potential toxicity .
Metabolic Pathways
BF-DPB is involved in various metabolic pathways, interacting with enzymes and cofactors that regulate metabolic flux and metabolite levels. For example, BF-DPB has been shown to influence the glycolytic pathway by modulating the activity of key enzymes, such as glucose-6-phosphate dehydrogenase and 6-phosphofructose kinase . These interactions alter the flow of metabolites through the pathway, leading to changes in energy production and cellular metabolism.
Transport and Distribution
Within cells and tissues, BF-DPB is transported and distributed through interactions with specific transporters and binding proteins. These interactions facilitate the localization and accumulation of BF-DPB in target tissues, enhancing its biochemical activity . For instance, BF-DPB has been shown to interact with ABC-transporter enzymes, which regulate its concentration within different tissue compartments and influence its overall efficacy .
Subcellular Localization
The subcellular localization of BF-DPB is critical for its activity and function. BF-DPB is directed to specific compartments or organelles within the cell through targeting signals and post-translational modifications . These localization mechanisms ensure that BF-DPB exerts its biochemical effects in the appropriate cellular context, enhancing its overall efficacy and minimizing potential off-target effects .
Preparation Methods
Synthetic Routes and Reaction Conditions
The synthesis of N,N’-Bis(9,9-dimethyl-9H-fluoren-2-yl)-N,N’-diphenylbenzidine typically involves multiple steps. One common method includes the following steps:
Formation of Key Intermediate: The key intermediate, 2-bromo-9-(2,5-dimethylphenyl)-9H-fluorene, is synthesized from 2-bromo-9-fluorenone through a sequence of reactions, including Grignard reaction, Et3SiH/BF3 reduction, or direct arylation of 2-bromo-9-fluorenol.
Alkylation and Cyclization: Alkylation at the C(9) position of 2-bromo-9-(2,5-dimethylphenyl)-9H-fluorene with methallyl chloride, followed by intramolecular Friedel-Crafts alkylation, leads to the formation of the key building block.
Industrial Production Methods
Industrial production methods for this compound are designed to be scalable and efficient. The process typically avoids chromatography, making it more suitable for large-scale production .
Chemical Reactions Analysis
Types of Reactions
N,N’-Bis(9,9-dimethyl-9H-fluoren-2-yl)-N,N’-diphenylbenzidine undergoes various chemical reactions, including:
Oxidation: This compound can be oxidized under specific conditions to form various oxidized derivatives.
Reduction: Reduction reactions can be performed to modify the electronic properties of the compound.
Substitution: Substitution reactions, particularly electrophilic aromatic substitution, are common for introducing different functional groups.
Common Reagents and Conditions
Oxidation: Common oxidizing agents include potassium permanganate and chromium trioxide.
Reduction: Reducing agents such as lithium aluminum hydride and sodium borohydride are used.
Substitution: Electrophilic aromatic substitution often involves reagents like sulfuric acid and nitric acid.
Major Products
The major products formed from these reactions depend on the specific conditions and reagents used. For example, oxidation can lead to the formation of quinones, while substitution can introduce various functional groups onto the aromatic rings .
Scientific Research Applications
N,N’-Bis(9,9-dimethyl-9H-fluoren-2-yl)-N,N’-diphenylbenzidine has a wide range of scientific research applications:
Organic Electronics: Used as a hole-transport material in OLEDs and organic solar cells due to its excellent electronic properties.
Photovoltaics: Plays a crucial role in the development of high-efficiency organic solar cells.
Material Science: Investigated for its potential in creating new materials with unique electronic and optical properties.
Chemical Sensors: Utilized in the development of sensors for detecting various chemical species.
Comparison with Similar Compounds
Similar Compounds
N,N-Bis(9,9-dimethyl-9H-fluoren-2-yl)aniline: Another compound with similar hole-transporting properties.
Bis(9,9-dimethyl-9H-fluoren-7-yl)amine: Known for its use in dye-sensitized solar cells.
Uniqueness
N,N’-Bis(9,9-dimethyl-9H-fluoren-2-yl)-N,N’-diphenylbenzidine stands out due to its high efficiency and stability in electronic applications. Its unique structural features, such as the presence of multiple fluorenyl groups, contribute to its superior performance compared to similar compounds .
Properties
IUPAC Name |
N-[4-[4-(N-(9,9-dimethylfluoren-2-yl)anilino)phenyl]phenyl]-9,9-dimethyl-N-phenylfluoren-2-amine | |
---|---|---|
Details | Computed by Lexichem TK 2.7.0 (PubChem release 2021.05.07) | |
Source | PubChem | |
URL | https://pubchem.ncbi.nlm.nih.gov | |
Description | Data deposited in or computed by PubChem | |
InChI |
InChI=1S/C54H44N2/c1-53(2)49-21-13-11-19-45(49)47-33-31-43(35-51(47)53)55(39-15-7-5-8-16-39)41-27-23-37(24-28-41)38-25-29-42(30-26-38)56(40-17-9-6-10-18-40)44-32-34-48-46-20-12-14-22-50(46)54(3,4)52(48)36-44/h5-36H,1-4H3 | |
Details | Computed by InChI 1.0.6 (PubChem release 2021.05.07) | |
Source | PubChem | |
URL | https://pubchem.ncbi.nlm.nih.gov | |
Description | Data deposited in or computed by PubChem | |
InChI Key |
VZYZZKOUCVXTOJ-UHFFFAOYSA-N | |
Details | Computed by InChI 1.0.6 (PubChem release 2021.05.07) | |
Source | PubChem | |
URL | https://pubchem.ncbi.nlm.nih.gov | |
Description | Data deposited in or computed by PubChem | |
Canonical SMILES |
CC1(C2=CC=CC=C2C3=C1C=C(C=C3)N(C4=CC=CC=C4)C5=CC=C(C=C5)C6=CC=C(C=C6)N(C7=CC=CC=C7)C8=CC9=C(C=C8)C1=CC=CC=C1C9(C)C)C | |
Details | Computed by OEChem 2.3.0 (PubChem release 2021.05.07) | |
Source | PubChem | |
URL | https://pubchem.ncbi.nlm.nih.gov | |
Description | Data deposited in or computed by PubChem | |
Molecular Formula |
C54H44N2 | |
Details | Computed by PubChem 2.1 (PubChem release 2021.05.07) | |
Source | PubChem | |
URL | https://pubchem.ncbi.nlm.nih.gov | |
Description | Data deposited in or computed by PubChem | |
Molecular Weight |
720.9 g/mol | |
Details | Computed by PubChem 2.1 (PubChem release 2021.05.07) | |
Source | PubChem | |
URL | https://pubchem.ncbi.nlm.nih.gov | |
Description | Data deposited in or computed by PubChem | |
Retrosynthesis Analysis
AI-Powered Synthesis Planning: Our tool employs the Template_relevance Pistachio, Template_relevance Bkms_metabolic, Template_relevance Pistachio_ringbreaker, Template_relevance Reaxys, Template_relevance Reaxys_biocatalysis model, leveraging a vast database of chemical reactions to predict feasible synthetic routes.
One-Step Synthesis Focus: Specifically designed for one-step synthesis, it provides concise and direct routes for your target compounds, streamlining the synthesis process.
Accurate Predictions: Utilizing the extensive PISTACHIO, BKMS_METABOLIC, PISTACHIO_RINGBREAKER, REAXYS, REAXYS_BIOCATALYSIS database, our tool offers high-accuracy predictions, reflecting the latest in chemical research and data.
Strategy Settings
Precursor scoring | Relevance Heuristic |
---|---|
Min. plausibility | 0.01 |
Model | Template_relevance |
Template Set | Pistachio/Bkms_metabolic/Pistachio_ringbreaker/Reaxys/Reaxys_biocatalysis |
Top-N result to add to graph | 6 |
Feasible Synthetic Routes
Disclaimer and Information on In-Vitro Research Products
Please be aware that all articles and product information presented on BenchChem are intended solely for informational purposes. The products available for purchase on BenchChem are specifically designed for in-vitro studies, which are conducted outside of living organisms. In-vitro studies, derived from the Latin term "in glass," involve experiments performed in controlled laboratory settings using cells or tissues. It is important to note that these products are not categorized as medicines or drugs, and they have not received approval from the FDA for the prevention, treatment, or cure of any medical condition, ailment, or disease. We must emphasize that any form of bodily introduction of these products into humans or animals is strictly prohibited by law. It is essential to adhere to these guidelines to ensure compliance with legal and ethical standards in research and experimentation.