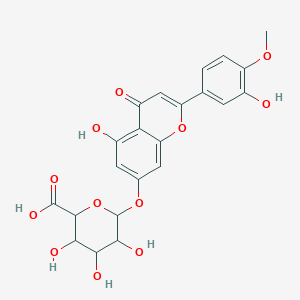
Hesperetin 7-O-b-D-Glucuronide
Overview
Description
Hesperetin 7-O-b-D-Glucuronide is a metabolite of hesperetin, a flavonoid found predominantly in citrus fruits. This compound is formed through the glucuronidation of hesperetin, a process that enhances its solubility and bioavailability. This compound is known for its various biological activities, including anti-inflammatory, antioxidant, and vasodilatory effects .
Mechanism of Action
Target of Action
DiosMetin 7-O-beta-D-Glucuronide is derived from Diosmin, a flavone glycoside . Diosmin is known for its potential involvement in human wellness and plays an important role in the treatment of various ailments .
Mode of Action
Diosmin, from which it is derived, is known to exhibit multiple desirable properties including anti-inflammatory, antioxidant, antidiabetic, antihyperlipidemic, and antifibrotic effects in different disease models . These effects suggest that DiosMetin 7-O-beta-D-Glucuronide may interact with its targets to modulate these pathways and induce these changes.
Biochemical Pathways
Diosmin and its derivatives are part of the class of flavonoids, secondary metabolites present in a huge number in plants and vegetables . Their biosynthesis in most species takes place following the general phenylpropanoid pathway and the flavonoid biosynthetic branch . The activation/block of the pathways associated with its utilization could be potential targets of action .
Pharmacokinetics
After oral administration, Diosmin is converted to Diosmetin, which is subsequently absorbed and esterified into glucuronide conjugates that are excreted in the urine . This process suggests that DiosMetin 7-O-beta-D-Glucuronide may have similar ADME (Absorption, Distribution, Metabolism, and Excretion) properties. Several studies report the low water solubility and bioavailability of diosmin , which may also apply to DiosMetin 7-O-beta-D-Glucuronide.
Result of Action
DiosMetin 7-O-beta-D-Glucuronide has been found to induce anti-inflammatory and antioxidant responses . The maximal effect is reached at a specific concentration, corresponding to the peak plasma concentration estimated after the oral intake of Diosmin . This suggests that DiosMetin 7-O-beta-D-Glucuronide may have similar molecular and cellular effects.
Preparation Methods
Synthetic Routes and Reaction Conditions
Hesperetin 7-O-b-D-Glucuronide can be synthesized through enzymatic hydrolysis of hesperidin, followed by glucuronidation. The enzymatic hydrolysis is typically carried out using naringinase, which converts hesperidin to hesperetin. The glucuronidation process involves the use of UDP-glucuronosyltransferase enzymes, which transfer glucuronic acid to hesperetin .
Industrial Production Methods
In industrial settings, the production of this compound often involves the use of immobilized enzyme catalysis platforms. For instance, immobilized rhamnosidase on Fe3O4@graphene oxide has been used to hydrolyze hesperidin into hesperetin, which is then glucuronidated to form this compound .
Chemical Reactions Analysis
Types of Reactions
Hesperetin 7-O-b-D-Glucuronide undergoes various chemical reactions, including:
Oxidation: This reaction can lead to the formation of quinones and other oxidized derivatives.
Reduction: Reduction reactions can convert the compound back to its aglycone form, hesperetin.
Substitution: Substitution reactions can occur at the hydroxyl groups, leading to the formation of different derivatives.
Common Reagents and Conditions
Oxidation: Common oxidizing agents include hydrogen peroxide and potassium permanganate.
Reduction: Reducing agents such as sodium borohydride are often used.
Substitution: Reagents like acetic anhydride and benzoyl chloride can be used for acetylation and benzoylation reactions, respectively.
Major Products Formed
Oxidation: Quinones and other oxidized derivatives.
Reduction: Hesperetin.
Substitution: Acetylated and benzoylated derivatives.
Scientific Research Applications
Hesperetin 7-O-b-D-Glucuronide has a wide range of scientific research applications:
Chemistry: Used as a model compound to study glucuronidation processes and enzyme kinetics.
Biology: Investigated for its role in cellular signaling pathways and its effects on gene expression.
Medicine: Explored for its potential therapeutic effects in treating hypertension, inflammation, and oxidative stress-related diseases.
Industry: Utilized in the formulation of functional foods and nutraceuticals due to its bioactive properties
Comparison with Similar Compounds
Similar Compounds
Hesperetin: The aglycone form of Hesperetin 7-O-b-D-Glucuronide, known for its antioxidant and anti-inflammatory properties.
Hesperidin: The glycoside form of hesperetin, commonly found in citrus fruits.
Hesperetin 7-O-Glucoside: Another glucoside derivative of hesperetin, with similar biological activities
Uniqueness
This compound is unique due to its enhanced solubility and bioavailability compared to its aglycone and glycoside counterparts. This makes it more effective in exerting its biological activities and easier to formulate in various pharmaceutical and nutraceutical products .
Properties
IUPAC Name |
3,4,5-trihydroxy-6-[5-hydroxy-2-(3-hydroxy-4-methoxyphenyl)-4-oxochromen-7-yl]oxyoxane-2-carboxylic acid | |
---|---|---|
Details | Computed by LexiChem 2.6.6 (PubChem release 2019.06.18) | |
Source | PubChem | |
URL | https://pubchem.ncbi.nlm.nih.gov | |
Description | Data deposited in or computed by PubChem | |
InChI |
InChI=1S/C22H20O12/c1-31-13-3-2-8(4-10(13)23)14-7-12(25)16-11(24)5-9(6-15(16)33-14)32-22-19(28)17(26)18(27)20(34-22)21(29)30/h2-7,17-20,22-24,26-28H,1H3,(H,29,30) | |
Details | Computed by InChI 1.0.5 (PubChem release 2019.06.18) | |
Source | PubChem | |
URL | https://pubchem.ncbi.nlm.nih.gov | |
Description | Data deposited in or computed by PubChem | |
InChI Key |
XCKMDTYMOHXUHG-UHFFFAOYSA-N | |
Details | Computed by InChI 1.0.5 (PubChem release 2019.06.18) | |
Source | PubChem | |
URL | https://pubchem.ncbi.nlm.nih.gov | |
Description | Data deposited in or computed by PubChem | |
Canonical SMILES |
COC1=C(C=C(C=C1)C2=CC(=O)C3=C(C=C(C=C3O2)OC4C(C(C(C(O4)C(=O)O)O)O)O)O)O | |
Details | Computed by OEChem 2.1.5 (PubChem release 2019.06.18) | |
Source | PubChem | |
URL | https://pubchem.ncbi.nlm.nih.gov | |
Description | Data deposited in or computed by PubChem | |
Molecular Formula |
C22H20O12 | |
Details | Computed by PubChem 2.1 (PubChem release 2019.06.18) | |
Source | PubChem | |
URL | https://pubchem.ncbi.nlm.nih.gov | |
Description | Data deposited in or computed by PubChem | |
Molecular Weight |
476.4 g/mol | |
Details | Computed by PubChem 2.1 (PubChem release 2021.05.07) | |
Source | PubChem | |
URL | https://pubchem.ncbi.nlm.nih.gov | |
Description | Data deposited in or computed by PubChem | |
CAS No. |
35110-20-4 | |
Record name | Diosmetin 7-O-beta-D-glucuronopyranoside | |
Source | Human Metabolome Database (HMDB) | |
URL | http://www.hmdb.ca/metabolites/HMDB0037452 | |
Description | The Human Metabolome Database (HMDB) is a freely available electronic database containing detailed information about small molecule metabolites found in the human body. | |
Explanation | HMDB is offered to the public as a freely available resource. Use and re-distribution of the data, in whole or in part, for commercial purposes requires explicit permission of the authors and explicit acknowledgment of the source material (HMDB) and the original publication (see the HMDB citing page). We ask that users who download significant portions of the database cite the HMDB paper in any resulting publications. | |
Retrosynthesis Analysis
AI-Powered Synthesis Planning: Our tool employs the Template_relevance Pistachio, Template_relevance Bkms_metabolic, Template_relevance Pistachio_ringbreaker, Template_relevance Reaxys, Template_relevance Reaxys_biocatalysis model, leveraging a vast database of chemical reactions to predict feasible synthetic routes.
One-Step Synthesis Focus: Specifically designed for one-step synthesis, it provides concise and direct routes for your target compounds, streamlining the synthesis process.
Accurate Predictions: Utilizing the extensive PISTACHIO, BKMS_METABOLIC, PISTACHIO_RINGBREAKER, REAXYS, REAXYS_BIOCATALYSIS database, our tool offers high-accuracy predictions, reflecting the latest in chemical research and data.
Strategy Settings
Precursor scoring | Relevance Heuristic |
---|---|
Min. plausibility | 0.01 |
Model | Template_relevance |
Template Set | Pistachio/Bkms_metabolic/Pistachio_ringbreaker/Reaxys/Reaxys_biocatalysis |
Top-N result to add to graph | 6 |
Feasible Synthetic Routes
Disclaimer and Information on In-Vitro Research Products
Please be aware that all articles and product information presented on BenchChem are intended solely for informational purposes. The products available for purchase on BenchChem are specifically designed for in-vitro studies, which are conducted outside of living organisms. In-vitro studies, derived from the Latin term "in glass," involve experiments performed in controlled laboratory settings using cells or tissues. It is important to note that these products are not categorized as medicines or drugs, and they have not received approval from the FDA for the prevention, treatment, or cure of any medical condition, ailment, or disease. We must emphasize that any form of bodily introduction of these products into humans or animals is strictly prohibited by law. It is essential to adhere to these guidelines to ensure compliance with legal and ethical standards in research and experimentation.