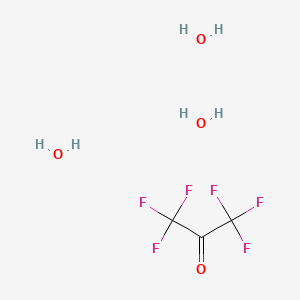
Hexafluoroacetone trihydrate
Overview
Description
Hexafluoroacetone trihydrate (HFA trihydrate, CAS: 34202-69-2) is a stable hydrate of hexafluoroacetone (HFA, CAS: 684-16-2), a highly reactive fluorinated ketone. The trihydrate form has the molecular formula C₃H₆F₆O₄ (derived from HFA + 3H₂O) and a molecular weight of 220.065 g/mol . It exists as a colorless, hygroscopic solid with a musty odor and a boiling point of 63°C . HFA trihydrate is commercially available at ≥97% purity and is widely used in organic synthesis, peptide stabilization, and polymer production .
Key applications include:
- Peptide research: Stabilizes intramolecular hydrogen bonds in aqueous solutions, outperforming trifluoroethanol (TFE) in conformational analysis .
- Silk fibroin processing: Dissolves fibroin for regenerated high-strength silk fibers, comparable to hexafluoroisopropanol (HFIP) .
- Organic synthesis: Serves as a precursor for quinolines, pipecolic acids, and bisphenol AF .
Preparation Methods
Synthetic Routes and Reaction Conditions
Hexafluoroacetone trihydrate can be synthesized through several methods:
Gas-phase exchange: This method involves the reaction of hexafluoropropylene oxide with water in the presence of a catalyst.
Oxidation: Hexafluoroacetone can be prepared by the oxidation of hexafluoropropylene oxide.
Isomerization: This process involves the isomerization of hexafluoro-1,2-epoxypropane in the presence of titanium oxide or fluorinated titanium oxide catalysts.
Industrial Production Methods
The industrial production of this compound typically involves the treatment of hexachloroacetone with hydrogen fluoride (HF) to produce hexafluoroacetone, which is then hydrated to form the trihydrate . Another method involves the rearrangement of hexafluoropropylene oxide to give hexafluoroacetone, followed by hydration .
Chemical Reactions Analysis
Carbonyl-Ene Reaction
HFA·3H₂O undergoes efficient carbonyl-ene reactions with alkenes under microwave or conventional heating, facilitated by molecular sieves. This reaction produces hexafluoroisopropanol (HFIP) derivatives in high yields (70–95%) .
Example Reaction:
Key Conditions:
Hydration/Dehydration Equilibrium
HFA·3H₂O exists in equilibrium with anhydrous HFA and water. The equilibrium constant () for hydration is , far exceeding acetone’s .
Dehydration Methods:
-
Acidic Dehydration : Treatment with concentrated sulfuric acid yields anhydrous HFA .
-
HF-Mediated Dehydration : Reacting with hydrogen fluoride (HF) produces a hexafluoroacetone-HF adduct, enabling anhydrous HFA recovery .
Reaction with Ammonia
HFA·3H₂O reacts with ammonia to form a hemiaminal intermediate, which dehydrates to an imine using phosphoryl chloride :
Nucleophilic Additions
The electron-deficient carbonyl group in HFA·3H₂O reacts with nucleophiles, forming lactones or amides. For example, hydroxy- and amine-substituted carboxylic acids yield cyclic esters or amides :
Applications : Synthesis of fluorinated polymers and pharmaceutical intermediates .
Photoredox-Catalyzed Deoxygenation
A novel method uses visible light and phosphine to deoxygenate HFA·3H₂O, enabling hydroxylpolyfluoroalkylation of electron-deficient alkenes :
Conditions :
Catalytic Hydrogenation
HFA·3H₂O is hydrogenated to HFIP, a precursor for the anesthetic sevoflurane. Continuous flow systems using Pd/C catalysts achieve 99% conversion and selectivity :
Optimized Parameters :
Acid-Base Reactions
HFA·3H₂O exhibits weak acidity () due to the electron-withdrawing CF₃ groups. It forms salts with strong bases (e.g., KOH) :
Comparative Reaction Data
Scientific Research Applications
Synthesis of Polymers
Hexafluoroacetone trihydrate is utilized in the synthesis of various polymers. Its unique fluorinated structure enhances the thermal stability and chemical resistance of the resulting materials. For instance, it has been used in the polymerization reactions with activated and non-activated aromatic monomers, leading to the development of fluorinated polymers with superior properties .
Organic Synthesis
HFA·3H₂O serves as a versatile reagent in organic synthesis. It acts as both a protecting and activating agent, particularly in peptide synthesis. Studies have shown that it can facilitate site-selective functionalization of amino acids and peptide mimetics, enabling more efficient synthetic pathways .
Kinetic Studies
Researchers have employed this compound to study the kinetics and thermodynamics of various chemical reactions. For example, it has been used in investigations involving linkage isomerization processes, contributing to a deeper understanding of reaction mechanisms in coordination chemistry .
Medicinal Chemistry
In medicinal chemistry, this compound has been explored as an intermediate in the synthesis of pharmaceutical compounds. Its reactivity allows for the introduction of fluorinated groups into drug candidates, which can enhance their biological activity and pharmacokinetic properties .
Environmental Applications
Recent studies have investigated the potential use of this compound in environmental chemistry, particularly in the degradation of pollutants. Its reactive nature may be harnessed to develop methods for breaking down hazardous substances .
Case Study 1: Polymerization Reactions
A study demonstrated the successful polymerization of this compound with various aromatic monomers, resulting in polymers with enhanced thermal properties. The research highlighted how the incorporation of fluorinated groups improved material stability under extreme conditions .
Case Study 2: Peptide Synthesis
In another investigation, hexafluoroacetone was utilized as a protecting group during solid-phase peptide synthesis. This approach allowed for selective functionalization and significantly improved yields compared to traditional methods .
Mechanism of Action
Hexafluoroacetone trihydrate exerts its effects primarily through its strong oxidizing properties. It acts as an electrophile, reacting vigorously with water to form a highly acidic hydrate . This reactivity allows it to participate in various chemical reactions, making it useful in synthetic chemistry and industrial applications.
Comparison with Similar Compounds
Comparison with Other HFA Hydrates
HFA reacts vigorously with water, forming hydrates of varying stoichiometry. The trihydrate is the most stable and commercially relevant form .
Comparison with Hexafluoroisopropanol (HFIP)
Both HFA trihydrate and HFIP (CAS: 920-66-1) are fluorinated solvents critical in biopolymer processing:
While both solvents dissolve fibroin effectively, HFA trihydrate is less volatile and offers superior safety profiles in handling .
Comparison with Trifluoroethanol (TFE)
HFA trihydrate and TFE (CAS: 75-89-8) are fluoroalcohols used to stabilize peptide structures:
HFA trihydrate’s gem-diol structure enhances its ability to stabilize compact peptide conformations, making it a preferred additive in structural biology .
Comparison with Halothane and Other Fluorinated Compounds
Unlike halothane (a fluorinated anesthetic), HFA trihydrate exhibits minimal protein binding and metabolic conversion:
Property | HFA Trihydrate | Halothane |
---|---|---|
Protein Binding | Negligible | Forms covalent adducts |
Metabolism | No significant metabolites | Hepatotoxic metabolites |
Toxicity | Low acute toxicity | High hepatotoxicity |
This low reactivity reduces risks in pharmaceutical applications, though stringent ventilation and PPE are still required during handling .
Market and Industrial Relevance
The global market for HFA hydrates is segmented by type (trihydrate vs. sesquihydrate) and application (medical, pesticides, organic chemicals). Key players include Shenzhen Capchem Technology and Sinochem Lantian . HFA trihydrate dominates due to its stability and versatility in synthesis.
Research Findings and Data Discrepancies
- Molecular Weight Conflict : reports 220.065 g/mol, while cites 386.0897 g/mol. This discrepancy likely stems from an error in , as the trihydrate formula (C₃H₆F₆O₄) aligns with the lower value .
- Safety : Despite low toxicity, HFA trihydrate requires precautions (e.g., ventilation, flame resistance) due to its hygroscopicity and decomposition risks at high temperatures .
Biological Activity
Hexafluoroacetone trihydrate (HFA·3H₂O) is a derivative of hexafluoroacetone, a highly reactive compound known for its industrial applications and biological activities. This article provides a comprehensive overview of the biological activity of this compound, including its toxicological effects, reproductive toxicity, and potential applications in biochemical research.
This compound is a hygroscopic solid that forms when hexafluoroacetone interacts with water. It has unique chemical properties due to the presence of fluorine atoms, which significantly influence its reactivity compared to its non-fluorinated counterparts like acetone. The equilibrium constant for the formation of HFA·3H₂O is notably high, indicating a strong tendency to hydrate in aqueous environments .
Acute Toxicity
The acute toxicity of this compound has been studied through various animal models. The following table summarizes key findings regarding its toxicity:
Endpoint | Value | Study Reference |
---|---|---|
Oral LD50 (rat) | 191 mg/kg | |
Inhalation LC50 (rat) | 275 ppm (3h exposure) | |
Dermal TDLo (rat) | 55 mg/kg |
These values indicate that HFA·3H₂O poses significant risks upon exposure, particularly through inhalation and ingestion.
Reproductive Toxicity
Research has highlighted reproductive toxicity associated with this compound. In studies involving pregnant rats, exposure during critical gestational periods led to adverse outcomes:
- Maternal Effects : Reduced weight gain and increased embryolethality were observed at high concentrations (≥6.9 mg/m³). The maternal No Observed Adverse Effect Level (NOAEL) was established at 0.76 mg/m³ .
- Fetal Effects : Significant reductions in fetal weights and increased rates of malformations were documented. Notably, external developmental variations such as anasarca and skeletal variations were prevalent in the high-concentration groups .
The mechanisms underlying the biological activity of this compound involve its interaction with cellular components:
- Lipid Metabolism Alteration : Hexafluoroacetone is thought to disrupt lipid metabolism, particularly affecting sterol metabolism, which may lead to testicular atrophy observed in male rats after prolonged exposure .
- Histopathological Changes : Histological evaluations have shown that HFA·3H₂O can cause severe atrophy in seminiferous tubules, affecting spermatogenesis and leading to oligospermia or aspermia .
Applications in Biochemical Research
This compound has also been explored for its utility in biochemical applications:
- Stabilization of Peptide Structures : Studies have demonstrated that HFA·3H₂O can stabilize secondary structures such as helices and hairpins in peptides, making it a valuable cosolvent for nuclear magnetic resonance (NMR) studies .
- Chemical Synthesis : It serves as a solvent for various chemical reactions, particularly in the synthesis of complex organic compounds due to its unique solvation properties .
Case Studies
Several case studies have investigated the effects of this compound on biological systems:
- Reproductive Study in Rats : A controlled study exposed pregnant rats to varying concentrations of HFA·3H₂O during gestation. Results indicated significant developmental toxicity at higher concentrations, affirming the compound's potential teratogenic effects .
- Testicular Toxicity Assessment : In male rats subjected to long-term inhalation exposure, notable changes in testicular morphology were observed, with recovery patterns analyzed post-exposure. The findings suggested partial restoration of spermatogenesis after a recovery period but highlighted lasting impacts on fertility potential .
Q & A
Basic Questions
Q. What are the critical safety protocols for handling HFA trihydrate in laboratory settings?
HFA trihydrate requires stringent safety measures due to its acute toxicity (oral, dermal, and inhalation), skin corrosion, and potential reproductive hazards . Key protocols include:
- Conducting experiments in a well-ventilated fume hood to minimize inhalation exposure .
- Wearing PPE: EN 166-compliant safety goggles, flame-resistant/waterproof clothing, and gloves tested for permeation resistance (e.g., nitrile or neoprene) .
- Avoiding static discharge and ignition sources, as HFA trihydrate decomposes at elevated temperatures (≥63°C) .
- Storing in airtight containers under inert gas (e.g., nitrogen) at room temperature to prevent hydration/dehydration reactions .
Q. How does HFA trihydrate stabilize peptide conformations in aqueous solutions?
HFA trihydrate acts as a strong structure-stabilizing solvent by promoting intramolecular hydrogen bonding in peptides, favoring α-helical and β-sheet conformations. This is attributed to its gem-diol structure (hexafluoropropan-2,2-diol), which disrupts bulk water networks while providing a low-dielectric environment that enhances hydrophobic interactions . Comparative studies show it outperforms trifluoroethanol (TFE) in stabilizing β-hairpin motifs due to stronger electrostatic effects .
Q. What are the key physicochemical properties of HFA trihydrate relevant to experimental design?
- Molecular formula : C₃F₆O·3H₂O (MW 220.07 g/mol) .
- Density : 1.579 g/cm³ at 20°C .
- Melting point : 18–21°C; decomposes above 63°C .
- Solubility : Miscible with polar solvents (e.g., water, DMSO) but immiscible with hydrocarbons .
- Hygroscopicity : Rapidly absorbs moisture, requiring anhydrous handling conditions for reactions sensitive to water .
Advanced Research Questions
Q. What analytical methods are suitable for detecting HFA trihydrate and its metabolites in biological systems?
- 19F-NMR spectroscopy : Identifies HFA trihydrate metabolites (e.g., hexafluoroacetone) in rat urine with high specificity, leveraging the fluorine atom's spin-½ nucleus for quantitative analysis .
- LC-MS/MS : Enables trace-level detection in microsomal assays (limit of quantification: 0.1 µg/mL) using negative-ion electrospray ionization .
- Circular Dichroism (CD) : Monitors conformational changes in peptides treated with HFA trihydrate by tracking ellipticity shifts at 190–250 nm .
Q. How does HFA trihydrate’s role as a metabolite of HFA-227 inform toxicological studies?
HFA trihydrate is a minor metabolite of 1,1,1,2,3,3,3-heptafluoropropane (HFA-227), an aerosol propellant. In vitro studies using rat and human liver microsomes show:
- Low biotransformation rates : Only 0.02% of HFA-227 converts to HFA trihydrate, even in CYP2E1-enriched microsomes .
- Negligible protein binding : Unlike halothane metabolites, HFA trihydrate does not form covalent adducts with human serum albumin or N-acetyl-L-lysine, minimizing immunotoxicity risks .
- No fluoride release : Unlike TFA derivatives, HFA trihydrate does not hydrolyze to free fluoride ions, reducing osteotoxicity concerns .
Q. What methodological advantages does HFA trihydrate offer over TFE in peptide studies?
- Enhanced β-sheet stabilization : HFA trihydrate’s bulkier fluorinated groups increase hydrophobic packing efficiency in β-hairpins compared to TFE .
- Reduced solvent perturbation : Lower volatility (bp 106–108°C) allows for stable long-term conformational analysis .
- Broader pH tolerance : Effective across pH 2–10 due to its inertness toward proton exchange, unlike TFE, which alters peptide charge states .
Q. How is HFA trihydrate utilized in synthesizing organometallic complexes?
HFA trihydrate reacts with imidazole 3-oxides to form crystalline complexes (e.g., 4b–4j) in >95% yield. The reaction proceeds via nucleophilic attack on the ketone carbonyl, followed by dehydration under mild conditions (CH₂Cl₂, 30 min, RT). The trihydrate’s stability ensures reproducible stoichiometry compared to anhydrous forms .
Q. How should researchers resolve contradictions in reported protein-binding data for HFA trihydrate?
Early concerns about protein adduct formation arose from structural analogies to halothane metabolites. However, 19F-NMR and microsomal assays confirm:
- No detectable binding : Fluorine signals remain unshifted in albumin interaction studies, ruling out covalent modification .
- Artifact mitigation : Use degassed buffers and anaerobic conditions to prevent oxidation byproducts during assays .
Q. What environmental persistence data exist for HFA trihydrate, and how should disposal be managed?
While direct environmental studies are limited, its high water solubility and low volatility (vapor pressure: 87.5 psi at 21°C) suggest moderate mobility in aquatic systems . Disposal must comply with UN 2552 regulations:
Properties
IUPAC Name |
1,1,1,3,3,3-hexafluoropropan-2-one;trihydrate | |
---|---|---|
Details | Computed by Lexichem TK 2.7.0 (PubChem release 2021.05.07) | |
Source | PubChem | |
URL | https://pubchem.ncbi.nlm.nih.gov | |
Description | Data deposited in or computed by PubChem | |
InChI |
InChI=1S/C3F6O.3H2O/c4-2(5,6)1(10)3(7,8)9;;;/h;3*1H2 | |
Details | Computed by InChI 1.0.6 (PubChem release 2021.05.07) | |
Source | PubChem | |
URL | https://pubchem.ncbi.nlm.nih.gov | |
Description | Data deposited in or computed by PubChem | |
InChI Key |
SNZAEUWCEHDROX-UHFFFAOYSA-N | |
Details | Computed by InChI 1.0.6 (PubChem release 2021.05.07) | |
Source | PubChem | |
URL | https://pubchem.ncbi.nlm.nih.gov | |
Description | Data deposited in or computed by PubChem | |
Canonical SMILES |
C(=O)(C(F)(F)F)C(F)(F)F.O.O.O | |
Details | Computed by OEChem 2.3.0 (PubChem release 2021.05.07) | |
Source | PubChem | |
URL | https://pubchem.ncbi.nlm.nih.gov | |
Description | Data deposited in or computed by PubChem | |
Molecular Formula |
C3H6F6O4 | |
Details | Computed by PubChem 2.1 (PubChem release 2021.05.07) | |
Source | PubChem | |
URL | https://pubchem.ncbi.nlm.nih.gov | |
Description | Data deposited in or computed by PubChem | |
Related CAS |
684-16-2 (Parent) | |
Record name | 2-Propanone, hexafluoro-, trihydrate | |
Source | ChemIDplus | |
URL | https://pubchem.ncbi.nlm.nih.gov/substance/?source=chemidplus&sourceid=0034202692 | |
Description | ChemIDplus is a free, web search system that provides access to the structure and nomenclature authority files used for the identification of chemical substances cited in National Library of Medicine (NLM) databases, including the TOXNET system. | |
DSSTOX Substance ID |
DTXSID201334105 | |
Record name | Hexafluoroacetone trihydrate | |
Source | EPA DSSTox | |
URL | https://comptox.epa.gov/dashboard/DTXSID201334105 | |
Description | DSSTox provides a high quality public chemistry resource for supporting improved predictive toxicology. | |
Molecular Weight |
220.07 g/mol | |
Details | Computed by PubChem 2.1 (PubChem release 2021.05.07) | |
Source | PubChem | |
URL | https://pubchem.ncbi.nlm.nih.gov | |
Description | Data deposited in or computed by PubChem | |
Physical Description |
Colorless liquid or melt; mp = 18-21 deg C; [Alfa Aesar MSDS] | |
Record name | Hexafluoroacetone trihydrate | |
Source | Haz-Map, Information on Hazardous Chemicals and Occupational Diseases | |
URL | https://haz-map.com/Agents/20995 | |
Description | Haz-Map® is an occupational health database designed for health and safety professionals and for consumers seeking information about the adverse effects of workplace exposures to chemical and biological agents. | |
Explanation | Copyright (c) 2022 Haz-Map(R). All rights reserved. Unless otherwise indicated, all materials from Haz-Map are copyrighted by Haz-Map(R). No part of these materials, either text or image may be used for any purpose other than for personal use. Therefore, reproduction, modification, storage in a retrieval system or retransmission, in any form or by any means, electronic, mechanical or otherwise, for reasons other than personal use, is strictly prohibited without prior written permission. | |
CAS No. |
34202-69-2, 13098-39-0 | |
Record name | 2-Propanone, hexafluoro-, trihydrate | |
Source | ChemIDplus | |
URL | https://pubchem.ncbi.nlm.nih.gov/substance/?source=chemidplus&sourceid=0034202692 | |
Description | ChemIDplus is a free, web search system that provides access to the structure and nomenclature authority files used for the identification of chemical substances cited in National Library of Medicine (NLM) databases, including the TOXNET system. | |
Record name | Hexafluoroacetone trihydrate | |
Source | EPA DSSTox | |
URL | https://comptox.epa.gov/dashboard/DTXSID201334105 | |
Description | DSSTox provides a high quality public chemistry resource for supporting improved predictive toxicology. | |
Record name | 2-Propanone, hexafluoro-, trihydrate | |
Source | European Chemicals Agency (ECHA) | |
URL | https://echa.europa.eu/information-on-chemicals | |
Description | The European Chemicals Agency (ECHA) is an agency of the European Union which is the driving force among regulatory authorities in implementing the EU's groundbreaking chemicals legislation for the benefit of human health and the environment as well as for innovation and competitiveness. | |
Explanation | Use of the information, documents and data from the ECHA website is subject to the terms and conditions of this Legal Notice, and subject to other binding limitations provided for under applicable law, the information, documents and data made available on the ECHA website may be reproduced, distributed and/or used, totally or in part, for non-commercial purposes provided that ECHA is acknowledged as the source: "Source: European Chemicals Agency, http://echa.europa.eu/". Such acknowledgement must be included in each copy of the material. ECHA permits and encourages organisations and individuals to create links to the ECHA website under the following cumulative conditions: Links can only be made to webpages that provide a link to the Legal Notice page. | |
Record name | 1,1,1,3,3,3-hexafluoropropan-2-one;trihydrate | |
Source | European Chemicals Agency (ECHA) | |
URL | https://echa.europa.eu/information-on-chemicals | |
Description | The European Chemicals Agency (ECHA) is an agency of the European Union which is the driving force among regulatory authorities in implementing the EU's groundbreaking chemicals legislation for the benefit of human health and the environment as well as for innovation and competitiveness. | |
Explanation | Use of the information, documents and data from the ECHA website is subject to the terms and conditions of this Legal Notice, and subject to other binding limitations provided for under applicable law, the information, documents and data made available on the ECHA website may be reproduced, distributed and/or used, totally or in part, for non-commercial purposes provided that ECHA is acknowledged as the source: "Source: European Chemicals Agency, http://echa.europa.eu/". Such acknowledgement must be included in each copy of the material. ECHA permits and encourages organisations and individuals to create links to the ECHA website under the following cumulative conditions: Links can only be made to webpages that provide a link to the Legal Notice page. | |
Synthesis routes and methods I
Procedure details
Synthesis routes and methods II
Procedure details
Synthesis routes and methods III
Procedure details
Retrosynthesis Analysis
AI-Powered Synthesis Planning: Our tool employs the Template_relevance Pistachio, Template_relevance Bkms_metabolic, Template_relevance Pistachio_ringbreaker, Template_relevance Reaxys, Template_relevance Reaxys_biocatalysis model, leveraging a vast database of chemical reactions to predict feasible synthetic routes.
One-Step Synthesis Focus: Specifically designed for one-step synthesis, it provides concise and direct routes for your target compounds, streamlining the synthesis process.
Accurate Predictions: Utilizing the extensive PISTACHIO, BKMS_METABOLIC, PISTACHIO_RINGBREAKER, REAXYS, REAXYS_BIOCATALYSIS database, our tool offers high-accuracy predictions, reflecting the latest in chemical research and data.
Strategy Settings
Precursor scoring | Relevance Heuristic |
---|---|
Min. plausibility | 0.01 |
Model | Template_relevance |
Template Set | Pistachio/Bkms_metabolic/Pistachio_ringbreaker/Reaxys/Reaxys_biocatalysis |
Top-N result to add to graph | 6 |
Feasible Synthetic Routes
Disclaimer and Information on In-Vitro Research Products
Please be aware that all articles and product information presented on BenchChem are intended solely for informational purposes. The products available for purchase on BenchChem are specifically designed for in-vitro studies, which are conducted outside of living organisms. In-vitro studies, derived from the Latin term "in glass," involve experiments performed in controlled laboratory settings using cells or tissues. It is important to note that these products are not categorized as medicines or drugs, and they have not received approval from the FDA for the prevention, treatment, or cure of any medical condition, ailment, or disease. We must emphasize that any form of bodily introduction of these products into humans or animals is strictly prohibited by law. It is essential to adhere to these guidelines to ensure compliance with legal and ethical standards in research and experimentation.