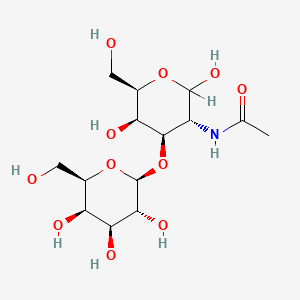
beta-D-Galp-(1->3)-D-GalpNAc
Overview
Description
beta-D-Galp-(1->3)-D-GalpNAc: is an amino disaccharide consisting of beta-D-galactose linked via a (1->3)-glycosidic bond to N-acetyl-D-galactosamine . This compound is a part of the glycan family and plays a significant role in various biological processes, including cell-cell recognition and signaling.
Preparation Methods
Synthetic Routes and Reaction Conditions
The synthesis of beta-D-Galp-(1->3)-D-GalpNAc typically involves the glycosylation of N-acetyl-D-galactosamine with beta-D-galactose. The reaction conditions often include the use of a glycosyl donor and acceptor, along with a catalyst to facilitate the formation of the glycosidic bond. Common catalysts include Lewis acids such as boron trifluoride etherate or trimethylsilyl trifluoromethanesulfonate.
Industrial Production Methods
Industrial production of beta-D-Galp-(1->3)-D-GalpNAc may involve enzymatic synthesis using glycosyltransferases. These enzymes catalyze the transfer of a sugar moiety from a donor molecule to an acceptor molecule, forming the desired glycosidic bond. This method is advantageous due to its high specificity and efficiency.
Chemical Reactions Analysis
Types of Reactions
beta-D-Galp-(1->3)-D-GalpNAc undergoes various chemical reactions, including:
Oxidation: The hydroxyl groups on the sugar moieties can be oxidized to form corresponding acids.
Reduction: The carbonyl group in N-acetyl-D-galactosamine can be reduced to form an alcohol.
Substitution: The hydroxyl groups can be substituted with other functional groups, such as halides or alkyl groups.
Common Reagents and Conditions
Oxidation: Reagents such as periodic acid or bromine water are commonly used.
Reduction: Sodium borohydride or lithium aluminum hydride are typical reducing agents.
Substitution: Halogenating agents like thionyl chloride or alkylating agents like methyl iodide are used.
Major Products Formed
Oxidation: Formation of uronic acids.
Reduction: Formation of alditols.
Substitution: Formation of halogenated or alkylated derivatives.
Scientific Research Applications
beta-D-Galp-(1->3)-D-GalpNAc has numerous applications in scientific research:
Chemistry: Used as a building block in the synthesis of complex glycans and glycoproteins.
Biology: Plays a role in cell-cell recognition and signaling, particularly in the immune system.
Medicine: Investigated for its potential in developing glycan-based therapeutics and vaccines.
Industry: Utilized in the production of glycosylated products, such as bioactive compounds and pharmaceuticals.
Mechanism of Action
The mechanism of action of beta-D-Galp-(1->3)-D-GalpNAc involves its interaction with specific receptors on the cell surface. These interactions can trigger various cellular responses, including signal transduction pathways that regulate immune responses and cell adhesion. The molecular targets include lectins and glycan-binding proteins that recognize the specific glycosidic linkage and structure of the compound.
Comparison with Similar Compounds
Similar Compounds
beta-D-Galp-(1->3)-beta-D-GlcpNAc: An amino disaccharide with a similar structure but different glycosidic linkage.
beta-D-Galp-(1->3)-beta-D-Galp-(1->4)-D-GlcpNAc: A trisaccharide with an additional galactose residue.
alpha-D-Galp-(1->3)-beta-D-Galp-(1->3)-beta-D-GlcpNAc-(1->2)-alpha-D-Manp: A tetrasaccharide with a more complex structure.
Uniqueness
beta-D-Galp-(1->3)-D-GalpNAc is unique due to its specific glycosidic linkage and the presence of N-acetyl-D-galactosamine. This structure imparts distinct biological properties, making it a valuable compound in various research and industrial applications.
Biological Activity
Introduction
Beta-D-Galp-(1->3)-D-GalpNAc is a trisaccharide composed of galactose and N-acetylgalactosamine units. This compound plays a significant role in various biological processes, particularly in cell recognition, immune response, and potential disease biomarkers. Understanding its biological activity is crucial for exploring its applications in diagnostics and therapeutics.
Structural Characteristics
Beta-D-Galp-(1->3)-D-GalpNAc features specific glycosidic linkages that define its structure and functionality:
- Composition : The compound consists of three sugar units:
- Beta-D-Galactopyranosyl (Galp)
- N-acetylgalactosamine (GalNAc)
- Linkages : The sugars are linked via:
- A (1→3) bond between the galactose and N-acetylgalactosamine.
This structural configuration allows beta-D-Galp-(1->3)-D-GalpNAc to participate in various biological interactions, particularly as a ligand for selectins, which are critical in cell adhesion processes.
Cell Recognition and Adhesion
Beta-D-Galp-(1->3)-D-GalpNAc has been identified as a ligand for selectins, which are cell adhesion molecules involved in immune responses. By binding to these selectins, the compound facilitates:
- Cell-Cell Interactions : This interaction is vital in processes such as leukocyte trafficking during inflammation.
- Immune Response Modulation : The compound may influence the migration of immune cells to sites of injury or infection.
Biomarker Potential
Research indicates that elevated levels of beta-D-Galp-(1->3)-D-GalpNAc can be detected in the blood and urine of patients with certain cancers, including:
- Gastric Cancer
- Colorectal Cancer
- Pancreatic Cancer
This suggests its potential utility as a biomarker for disease diagnosis and progression monitoring. However, further studies are required to validate these findings and elucidate the underlying mechanisms.
Interaction with Pathogens
The compound may also play a role in the interaction with pathogens. Some studies suggest that beta-D-Galp-(1->3)-D-GalpNAc could be involved in:
- Bacterial Adhesion : Certain bacteria may exploit this trisaccharide to adhere to host tissues, facilitating infection.
Case Studies
-
Cancer Biomarkers :
- A study demonstrated that patients with elevated levels of beta-D-Galp-(1->3)-D-GalpNAc had a higher incidence of colorectal cancer compared to controls. This was attributed to the compound's role in tumor progression and metastasis.
-
Inflammatory Responses :
- In models of acute inflammation, blocking the interaction between beta-D-Galp-(1->3)-D-GalpNAc and selectins resulted in reduced leukocyte infiltration, highlighting its role in mediating inflammatory responses.
Synthesis and Applications
The synthesis of beta-D-Galp-(1->3)-D-GalpNAc has been achieved through various methods, including:
- Chemical Synthesis : Utilizing glycosylation reactions under controlled conditions.
- Enzymatic Methods : Employing glycosyltransferases for more specific linkage formation.
These synthetic approaches not only facilitate research but also enable potential therapeutic applications, such as designing glycan-based drugs or vaccines.
Comparative Analysis
Compound Name | Structural Features | Unique Aspects |
---|---|---|
Beta-D-Galp-(1->4)-Beta-D-GlcpNAc | Contains a (1→4) linkage | Important for blood group antigens |
Alpha-D-Galp-(1->4)-Beta-D-Galp | Different linkage type | Commonly found in human blood group substances |
Beta-D-Manp-(1->4)-Beta-D-GlcpNAc | Mannose residue instead of galactose | Key component of certain bacterial polysaccharides |
This table illustrates the uniqueness of beta-D-Galp-(1->3)-D-GalpNAc due to its specific glycosidic linkages and biological roles compared to structurally similar compounds.
Properties
IUPAC Name |
N-[(3R,4R,5R,6R)-2,5-dihydroxy-6-(hydroxymethyl)-4-[(2R,3R,4S,5R,6R)-3,4,5-trihydroxy-6-(hydroxymethyl)oxan-2-yl]oxyoxan-3-yl]acetamide | |
---|---|---|
Details | Computed by Lexichem TK 2.7.0 (PubChem release 2021.05.07) | |
Source | PubChem | |
URL | https://pubchem.ncbi.nlm.nih.gov | |
Description | Data deposited in or computed by PubChem | |
InChI |
InChI=1S/C14H25NO11/c1-4(18)15-7-12(9(20)6(3-17)24-13(7)23)26-14-11(22)10(21)8(19)5(2-16)25-14/h5-14,16-17,19-23H,2-3H2,1H3,(H,15,18)/t5-,6-,7-,8+,9+,10+,11-,12-,13?,14+/m1/s1 | |
Details | Computed by InChI 1.0.6 (PubChem release 2021.05.07) | |
Source | PubChem | |
URL | https://pubchem.ncbi.nlm.nih.gov | |
Description | Data deposited in or computed by PubChem | |
InChI Key |
HMQPEDMEOBLSQB-UITYFYQISA-N | |
Details | Computed by InChI 1.0.6 (PubChem release 2021.05.07) | |
Source | PubChem | |
URL | https://pubchem.ncbi.nlm.nih.gov | |
Description | Data deposited in or computed by PubChem | |
Canonical SMILES |
CC(=O)NC1C(C(C(OC1O)CO)O)OC2C(C(C(C(O2)CO)O)O)O | |
Details | Computed by OEChem 2.3.0 (PubChem release 2021.05.07) | |
Source | PubChem | |
URL | https://pubchem.ncbi.nlm.nih.gov | |
Description | Data deposited in or computed by PubChem | |
Isomeric SMILES |
CC(=O)N[C@@H]1[C@H]([C@H]([C@H](OC1O)CO)O)O[C@H]2[C@@H]([C@H]([C@H]([C@H](O2)CO)O)O)O | |
Details | Computed by OEChem 2.3.0 (PubChem release 2021.05.07) | |
Source | PubChem | |
URL | https://pubchem.ncbi.nlm.nih.gov | |
Description | Data deposited in or computed by PubChem | |
Molecular Formula |
C14H25NO11 | |
Details | Computed by PubChem 2.1 (PubChem release 2021.05.07) | |
Source | PubChem | |
URL | https://pubchem.ncbi.nlm.nih.gov | |
Description | Data deposited in or computed by PubChem | |
DSSTOX Substance ID |
DTXSID80943264 | |
Record name | Galacto-N-biose | |
Source | EPA DSSTox | |
URL | https://comptox.epa.gov/dashboard/DTXSID80943264 | |
Description | DSSTox provides a high quality public chemistry resource for supporting improved predictive toxicology. | |
Molecular Weight |
383.35 g/mol | |
Details | Computed by PubChem 2.1 (PubChem release 2021.05.07) | |
Source | PubChem | |
URL | https://pubchem.ncbi.nlm.nih.gov | |
Description | Data deposited in or computed by PubChem | |
CAS No. |
20972-29-6 | |
Record name | 2-(Acetylamino)-2-deoxy-3-O-β-D-galactopyranosyl-D-galactopyranose | |
Source | CAS Common Chemistry | |
URL | https://commonchemistry.cas.org/detail?cas_rn=20972-29-6 | |
Description | CAS Common Chemistry is an open community resource for accessing chemical information. Nearly 500,000 chemical substances from CAS REGISTRY cover areas of community interest, including common and frequently regulated chemicals, and those relevant to high school and undergraduate chemistry classes. This chemical information, curated by our expert scientists, is provided in alignment with our mission as a division of the American Chemical Society. | |
Explanation | The data from CAS Common Chemistry is provided under a CC-BY-NC 4.0 license, unless otherwise stated. | |
Record name | Galacto-N-biose | |
Source | EPA DSSTox | |
URL | https://comptox.epa.gov/dashboard/DTXSID80943264 | |
Description | DSSTox provides a high quality public chemistry resource for supporting improved predictive toxicology. | |
Q1: What is the structure of the TF epitope?
A1: The TF epitope is a disaccharide composed of galactose (Gal) and N-acetylgalactosamine (GalNAc) linked via a beta-(1->3) glycosidic bond. Its chemical name is beta-D-Galp-(1->3)-D-GalpNAc.
Q2: How does the TF epitope interact with other molecules?
A2: The TF epitope is recognized by various carbohydrate-binding proteins, including lectins like galectin-1 [, , , ]. This interaction can lead to downstream effects like cell adhesion, apoptosis, and modulation of tumor cell behavior.
Q3: Is the TF epitope found in healthy tissues?
A3: While primarily considered a tumor-associated antigen, the TF epitope is also expressed in normal tissues, particularly during specific developmental stages or under certain physiological conditions. For example, it's found in the human placenta [, ] and on human oocytes [].
Q4: How is the TF epitope linked to cancer?
A4: The TF epitope is overexpressed in various cancers, including epithelial carcinomas [, , , , ]. This aberrant glycosylation is linked to tumor progression, metastasis, and poor prognosis.
Q5: Can the TF epitope be targeted for cancer therapy?
A5: The TF epitope's overexpression in cancer makes it a potential target for therapies like immunotherapy. Synthetic glycoconjugates mimicking the TF epitope have shown promise as anti-cancer vaccines by stimulating immune responses against tumors [].
Q6: How does TF contribute to tumor metastasis?
A6: Elevated levels of galectins, such as galectin-2, -3, -4, and -8, are found in cancer patients, particularly those with metastases []. These galectins bind to the TF epitope on cancer-associated mucin 1 (MUC1), promoting cancer cell adhesion to blood vessels and potentially facilitating metastasis [].
Q7: What are C-linked disaccharide analogs of the TF epitope?
A7: These are synthetic analogs where the oxygen atom in the glycosidic bond is replaced with a methylene (-CH2-) group [, ]. This modification increases stability against enzymatic degradation, making them potentially more suitable for therapeutic applications like vaccine development.
Q8: What are the challenges in developing TF-based vaccines?
A8: One challenge is the potential for cross-reactivity with normal tissues expressing the TF epitope []. Additionally, optimizing the immunogenicity and efficacy of these vaccines requires further research.
Q9: How does Schistosoma mansoni infection relate to the TF epitope?
A9: Mice infected with the parasite Schistosoma mansoni develop antibodies against the TF epitope due to its presence in the parasite []. This finding highlights the potential for cross-reactivity between parasite antigens and human tumor markers.
Q10: What is the role of human monoclonal antibodies targeting the TF epitope?
A10: Researchers have generated human monoclonal antibodies that specifically bind to the TF antigen []. These antibodies hold potential for diagnostic and therapeutic applications, particularly in the context of cancer.
Disclaimer and Information on In-Vitro Research Products
Please be aware that all articles and product information presented on BenchChem are intended solely for informational purposes. The products available for purchase on BenchChem are specifically designed for in-vitro studies, which are conducted outside of living organisms. In-vitro studies, derived from the Latin term "in glass," involve experiments performed in controlled laboratory settings using cells or tissues. It is important to note that these products are not categorized as medicines or drugs, and they have not received approval from the FDA for the prevention, treatment, or cure of any medical condition, ailment, or disease. We must emphasize that any form of bodily introduction of these products into humans or animals is strictly prohibited by law. It is essential to adhere to these guidelines to ensure compliance with legal and ethical standards in research and experimentation.