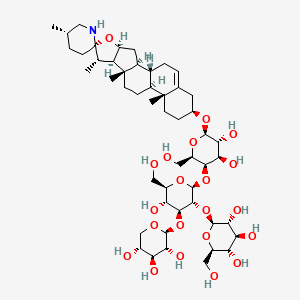
dehydrotomatine
Overview
Description
Dehydrotomatine is a steroidal glycoalkaloid found predominantly in the green fruits, leaves, and flowers of the tomato plant (Solanum lycopersicum). It serves as a defensive compound against various pathogens and predators, including bacteria, fungi, insects, and animals . The aglycone of this compound is dehydrotomatidine, which features a double bond between carbon atoms 5 and 6, distinguishing it from its counterpart, tomatidine .
Mechanism of Action
Target of Action
Dehydrotomatine, a steroidal glycoalkaloid (SGA), primarily targets bacteria, fungi, insects, and animals . It is found in mature green fruits, leaves, and flowers of tomatoes and functions as a defensive compound against these pathogens and predators .
Mode of Action
It is known to inhibit the growth of certain strains of protozoan parasites, bacteria, and fungi . It is suggested that this compound interacts with its targets, leading to their growth inhibition .
Biochemical Pathways
This compound is involved in the α-tomatine biosynthesis pathway in tomatoes . A key enzyme, Sl3βHSD1, catalyzes the C3 oxidation of dehydrotomatidine (a precursor of this compound) to form tomatid-4-en-3-one and also catalyzes the NADH-dependent C3 reduction of a 3-ketosteroid (tomatid-3-one) to form tomatidine . This conversion process is crucial in the biosynthesis of α-tomatine .
Pharmacokinetics
It is known that this compound is present in commercial tomatine preparations and those extracted from green tomatoes and tomato leaves .
Result of Action
Action Environment
The action of this compound can be influenced by environmental factors. For instance, the concentration of this compound in tomato plants can vary depending on the maturity of the plant parts. It has been observed that leaves, stems, and immature green tomato peels and fruit, all containing tomatine, were more effective as inhibitors than those prepared from yellow and red tomato peels which lack tomatine .
Biochemical Analysis
Biochemical Properties
The aglycone of dehydrotomatine is dehydrotomatidine . Biochemical analysis of the recombinant Sl3βHSD1 protein revealed that Sl3βHSD1 catalyzes the C3 oxidation of dehydrotomatidine to form tomatid-4-en-3-one and also catalyzes the NADH-dependent C3 reduction of a 3-ketosteroid (tomatid-3-one) to form tomatidine .
Cellular Effects
The cellular effects of this compound are primarily related to its role as a defensive compound. It is known to have antimicrobial and insecticidal properties, helping to protect the plant from various pathogens and pests .
Molecular Mechanism
The molecular mechanism of this compound involves the conversion of dehydrotomatidine to tomatidine via four reaction steps: C3 oxidation, isomerization, C5 reduction, and C3 reduction . The enzyme Sl3βHSD1 is involved in these conversion processes .
Temporal Effects in Laboratory Settings
It is known that the compound accumulates in mature green fruits, leaves, and flowers of tomatoes .
Dosage Effects in Animal Models
It is known that steroidal glycoalkaloids, including this compound, can have toxic effects at high doses .
Metabolic Pathways
This compound is involved in the steroidal glycoalkaloid biosynthesis pathway. The enzyme Sl3βHSD1 plays a key role in this pathway, catalyzing the conversion of dehydrotomatidine to tomatidine .
Transport and Distribution
It is known that the compound accumulates in mature green fruits, leaves, and flowers of tomatoes .
Subcellular Localization
As a steroidal glycoalkaloid, it is likely to be found in the vacuoles of plant cells, where these compounds are typically stored .
Preparation Methods
Synthetic Routes and Reaction Conditions: Dehydrotomatine is typically extracted from tomato plants. The extraction process involves harvesting the green fruits, leaves, and flowers, followed by freeze-drying and grinding the plant material. The powdered material is then subjected to high-performance liquid chromatography (HPLC) to isolate this compound .
Industrial Production Methods: Industrial production of this compound involves large-scale cultivation of tomato plants, followed by mechanical harvesting and processing. The plant material is processed to extract this compound using solvent extraction methods, followed by purification using chromatographic techniques .
Chemical Reactions Analysis
Types of Reactions: Dehydrotomatine undergoes various chemical reactions, including oxidation, reduction, and isomerization. The compound can be oxidized at the C3 position to form tomatid-4-en-3-one and subsequently reduced to tomatidine .
Common Reagents and Conditions:
Oxidation: Catalyzed by short-chain alcohol dehydrogenase/reductase enzymes.
Reduction: NADH-dependent reduction of 3-ketosteroids.
Major Products:
Tomatid-4-en-3-one: Formed through C3 oxidation.
Tomatidine: Formed through NADH-dependent reduction of tomatid-3-one.
Scientific Research Applications
Dehydrotomatine has several scientific research applications:
Chemistry: Used as a model compound to study glycoalkaloid biosynthesis and metabolism.
Biology: Investigated for its role in plant defense mechanisms and interactions with pathogens.
Medicine: Explored for its potential antimicrobial, anti-inflammatory, and anticancer properties.
Industry: Utilized in the development of natural pesticides and biocontrol agents.
Comparison with Similar Compounds
- α-Tomatine
- α-Solanine
- α-Chaconine
Dehydrotomatine’s unique structural features and diverse biological activities make it a valuable compound for scientific research and industrial applications.
Properties
IUPAC Name |
(2S,3R,4S,5S,6R)-2-[(2S,3R,4S,5R,6R)-2-[(2R,3R,4R,5R,6R)-4,5-dihydroxy-2-(hydroxymethyl)-6-[(1S,2S,4S,5'S,6S,7S,8R,9S,12S,13R,16S)-5',7,9,13-tetramethylspiro[5-oxapentacyclo[10.8.0.02,9.04,8.013,18]icos-18-ene-6,2'-piperidine]-16-yl]oxyoxan-3-yl]oxy-5-hydroxy-6-(hydroxymethyl)-4-[(2S,3R,4S,5R)-3,4,5-trihydroxyoxan-2-yl]oxyoxan-3-yl]oxy-6-(hydroxymethyl)oxane-3,4,5-triol | |
---|---|---|
Details | Computed by Lexichem TK 2.7.0 (PubChem release 2021.05.07) | |
Source | PubChem | |
URL | https://pubchem.ncbi.nlm.nih.gov | |
Description | Data deposited in or computed by PubChem | |
InChI |
InChI=1S/C50H81NO21/c1-20-7-12-50(51-15-20)21(2)32-28(72-50)14-26-24-6-5-22-13-23(8-10-48(22,3)25(24)9-11-49(26,32)4)65-45-40(63)37(60)41(31(18-54)68-45)69-47-43(71-46-39(62)36(59)34(57)29(16-52)66-46)42(35(58)30(17-53)67-47)70-44-38(61)33(56)27(55)19-64-44/h5,20-21,23-47,51-63H,6-19H2,1-4H3/t20-,21-,23-,24+,25-,26-,27+,28-,29+,30+,31+,32-,33-,34+,35+,36-,37+,38+,39+,40+,41-,42-,43+,44-,45+,46-,47-,48-,49-,50-/m0/s1 | |
Details | Computed by InChI 1.0.6 (PubChem release 2021.05.07) | |
Source | PubChem | |
URL | https://pubchem.ncbi.nlm.nih.gov | |
Description | Data deposited in or computed by PubChem | |
InChI Key |
BYMOGFTUZUEFHY-SIUCFGLGSA-N | |
Details | Computed by InChI 1.0.6 (PubChem release 2021.05.07) | |
Source | PubChem | |
URL | https://pubchem.ncbi.nlm.nih.gov | |
Description | Data deposited in or computed by PubChem | |
Canonical SMILES |
CC1CCC2(C(C3C(O2)CC4C3(CCC5C4CC=C6C5(CCC(C6)OC7C(C(C(C(O7)CO)OC8C(C(C(C(O8)CO)O)OC9C(C(C(CO9)O)O)O)OC2C(C(C(C(O2)CO)O)O)O)O)O)C)C)C)NC1 | |
Details | Computed by OEChem 2.3.0 (PubChem release 2021.05.07) | |
Source | PubChem | |
URL | https://pubchem.ncbi.nlm.nih.gov | |
Description | Data deposited in or computed by PubChem | |
Isomeric SMILES |
C[C@H]1CC[C@]2([C@H]([C@H]3[C@@H](O2)C[C@@H]4[C@@]3(CC[C@H]5[C@H]4CC=C6[C@@]5(CC[C@@H](C6)O[C@H]7[C@@H]([C@H]([C@H]([C@H](O7)CO)O[C@H]8[C@@H]([C@H]([C@@H]([C@H](O8)CO)O)O[C@H]9[C@@H]([C@H]([C@@H](CO9)O)O)O)O[C@H]2[C@@H]([C@H]([C@@H]([C@H](O2)CO)O)O)O)O)O)C)C)C)NC1 | |
Details | Computed by OEChem 2.3.0 (PubChem release 2021.05.07) | |
Source | PubChem | |
URL | https://pubchem.ncbi.nlm.nih.gov | |
Description | Data deposited in or computed by PubChem | |
Molecular Formula |
C50H81NO21 | |
Details | Computed by PubChem 2.1 (PubChem release 2021.05.07) | |
Source | PubChem | |
URL | https://pubchem.ncbi.nlm.nih.gov | |
Description | Data deposited in or computed by PubChem | |
DSSTOX Substance ID |
DTXSID001317151 | |
Record name | Dehydrotomatine | |
Source | EPA DSSTox | |
URL | https://comptox.epa.gov/dashboard/DTXSID001317151 | |
Description | DSSTox provides a high quality public chemistry resource for supporting improved predictive toxicology. | |
Molecular Weight |
1032.2 g/mol | |
Details | Computed by PubChem 2.1 (PubChem release 2021.05.07) | |
Source | PubChem | |
URL | https://pubchem.ncbi.nlm.nih.gov | |
Description | Data deposited in or computed by PubChem | |
CAS No. |
157604-98-3 | |
Record name | Dehydrotomatine | |
Source | CAS Common Chemistry | |
URL | https://commonchemistry.cas.org/detail?cas_rn=157604-98-3 | |
Description | CAS Common Chemistry is an open community resource for accessing chemical information. Nearly 500,000 chemical substances from CAS REGISTRY cover areas of community interest, including common and frequently regulated chemicals, and those relevant to high school and undergraduate chemistry classes. This chemical information, curated by our expert scientists, is provided in alignment with our mission as a division of the American Chemical Society. | |
Explanation | The data from CAS Common Chemistry is provided under a CC-BY-NC 4.0 license, unless otherwise stated. | |
Record name | Dehydrotomatine | |
Source | EPA DSSTox | |
URL | https://comptox.epa.gov/dashboard/DTXSID001317151 | |
Description | DSSTox provides a high quality public chemistry resource for supporting improved predictive toxicology. | |
A: Dehydrotomatine has a molecular formula of C50H83NO21 and a molecular weight of 1034.2 g/mol. [, , ]
A: this compound and α-tomatine share the same tetrasaccharide side chain (lycotetraose) attached to a steroidal aglycone. The key difference lies in the aglycone structure: this compound possesses a double bond between carbon atoms 5 and 6 in its aglycone (tomatidenol), while α-tomatine's aglycone (tomatidine) lacks this double bond. [, , , , ]
A: Various techniques are used to characterize this compound, including:* High-performance liquid chromatography (HPLC) [, , , ]* Mass spectrometry (MS) [, , , ] * Tandem mass spectrometry (MS/MS) [, ]* Nuclear Magnetic Resonance (NMR) []* Gas Chromatography-Mass Spectrometry (GC-MS) []
A: this compound biosynthesis in tomato plants follows a complex pathway involving multiple enzymatic steps. The process begins with cholesterol and ultimately leads to the formation of dehydrotomatidine, the aglycone of this compound. This aglycone is then glycosylated with a tetrasaccharide moiety to form this compound. [, , , ]
A: Research suggests that the biosynthesis of these glycoalkaloids might be under separate genetic control. This is supported by the observation that the ratio of α-tomatine to this compound varies significantly across different tomato plant tissues. []
A: this compound is present in various parts of the tomato plant, including fruits (both green and red), leaves, and other vegetative tissues. [, , , , ]
A: While research on this compound is ongoing, some studies suggest it may possess the following biological activities:* Antitumor Potential: Studies have shown that this compound, in combination with α-tomatine, exhibits cytotoxic effects against various cancer cell lines in vitro, including breast, colon, liver, and stomach cancer cells. [, , ]* Antihypertensive Effects: this compound found in green tomato extracts, along with other bioactive compounds, has been associated with reduced blood pressure in spontaneously hypertensive rats. []* Anti-fungal activity: Research suggests that this compound, along with α-tomatine, might contribute to the plant's defense mechanisms against fungal pathogens. []
A: The biological activities exhibited by this compound suggest potential applications in various fields:* Medicine: Its antitumor and antihypertensive properties warrant further investigation for potential use in developing novel therapeutic agents. [, , ]* Agriculture: Understanding the role of this compound in plant defense mechanisms could contribute to developing pest-resistant tomato varieties. [, ]
A: this compound belongs to the glycoalkaloid family, some members of which can be toxic to humans at high concentrations. While this compound is found in tomatoes, its levels are generally low, especially in ripe fruits. [] Further research is needed to fully understand the potential toxicity of this compound in humans, particularly at high concentrations.
Disclaimer and Information on In-Vitro Research Products
Please be aware that all articles and product information presented on BenchChem are intended solely for informational purposes. The products available for purchase on BenchChem are specifically designed for in-vitro studies, which are conducted outside of living organisms. In-vitro studies, derived from the Latin term "in glass," involve experiments performed in controlled laboratory settings using cells or tissues. It is important to note that these products are not categorized as medicines or drugs, and they have not received approval from the FDA for the prevention, treatment, or cure of any medical condition, ailment, or disease. We must emphasize that any form of bodily introduction of these products into humans or animals is strictly prohibited by law. It is essential to adhere to these guidelines to ensure compliance with legal and ethical standards in research and experimentation.