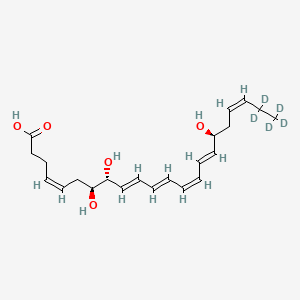
7S,8R,17S-trihydroxy-4Z,9E,11E,13Z,15E,19Z-21,21',22,22,22-d5docosahexaenoicacid
Overview
Description
Resolvin D1-d5 (RvD1-d5): is a deuterium-labeled derivative of Resolvin D1 (RvD1) . RvD1 is an endogenous pro-resolving mediator of inflammation, derived from omega-3 docosahexaenoic acid during the resolution phase of acute inflammation . Its primary role lies in regulating inflammation and promoting tissue repair.
Mechanism of Action
Target of Action
Resolvin D1-d5, also known as 7S,8R,17S-trihydroxy-4Z,9E,11E,13Z,15E,19Z-21,21’,22,22,22-d5docosahexaenoic acid, primarily targets neutrophils and macrophages . These immune cells play a crucial role in the inflammatory response. Neutrophils are the first responders to inflammation, migrating to the site of injury or infection, while macrophages are responsible for phagocytosis of apoptotic cells and debris .
Mode of Action
Resolvin D1-d5 interacts with its targets by regulating actin polymerization, which blocks proinflammatory neutrophil migration . It also reduces TNF-α–mediated inflammation in macrophages and enhances the phagocytosis of apoptotic cells by macrophages . This interaction results in a reduction of inflammation and promotion of the resolution process .
Biochemical Analysis
Biochemical Properties
Resolvin D1-d5 has been observed to trigger the process of inflammation resolution, thereby reinstating the homeostasis of the inflammatory response . It interacts with various enzymes, proteins, and other biomolecules, playing a significant role in the regulation of the inflammatory response . For instance, it has been shown to control downstream miR-30e-5p to increase Treg and reduce the differentiation of Th17, repairing the imbalance in the Treg/Th17 ratio .
Cellular Effects
Resolvin D1-d5 has profound effects on various types of cells and cellular processes. It influences cell function by impacting cell signaling pathways, gene expression, and cellular metabolism . For example, it has been shown to significantly inhibit the Th17 cells’ differentiation and proliferation . Moreover, it has been found to reduce TNF-α–mediated inflammation in macrophages and enhance phagocytosis of apoptotic cells by macrophages .
Molecular Mechanism
At the molecular level, Resolvin D1-d5 exerts its effects through various mechanisms. It has been shown to reduce inflammation by suppressing the Stat1-Cxcl10 signaling pathway in macrophages . Furthermore, it prevents hepatocyte death by alleviating ER stress-mediated apoptosis .
Temporal Effects in Laboratory Settings
In laboratory settings, the effects of Resolvin D1-d5 change over time. It has been observed that Resolvin D1-d5 treatment prevents the more pronounced anxious-like and reduced the depressive-like behaviors of experimental-T1DM animals and significantly improves the plasma glucose levels .
Dosage Effects in Animal Models
The effects of Resolvin D1-d5 vary with different dosages in animal models. For instance, in a mouse model of myocardial ischemia repefusuin (MI/R) injury, intravenous injection of Resolvin D1-d5 resulted in the enrichment of Resolvin D1-d5 in the injured area, which in turn promotes clearance of dead cells, production of specialized pro-resolving mediators (SPMs), and angiogenesis during injury repair, effectively improving cardiac function .
Metabolic Pathways
Resolvin D1-d5 is involved in various metabolic pathways. It is synthesized through the stereo-selective transformation of essential fatty acids, resulting in molecules dynamically modulated during inflammation and possessing strong immunoregulatory properties .
Subcellular Localization
It is known that Resolvin D1-d5 plays a key role in preventing bone resorption and other pathophysiological changes associated with arthritis .
Preparation Methods
Synthetic Routes:
The synthesis of RvD1-d5 involves incorporating deuterium atoms into the Resolvin D1 structure. Specific synthetic routes are not widely documented, but deuterium substitution can occur at various positions within the molecule.
Industrial Production:
Industrial-scale production methods for RvD1-d5 are not well-established due to its specialized use as an internal standard in analytical techniques.
Chemical Reactions Analysis
RvD1-d5 is a lipid mediator, and its chemical behavior is influenced by its structure. Here are some key aspects:
Reactions: RvD1-d5 may undergo various reactions, including oxidation, reduction, and substitution. detailed studies on its reactivity are limited.
Common Reagents and Conditions: Specific reagents and conditions for RvD1-d5 reactions are not widely reported.
Major Products: The major products resulting from RvD1-d5 reactions would depend on the specific reaction type and conditions.
Scientific Research Applications
RvD1-d5 has garnered interest in several scientific fields:
Inflammation Research: As an anti-inflammatory mediator, RvD1-d5 contributes to resolving inflammation by modulating neutrophil migration and reducing TNF-α-mediated inflammation in macrophages.
Immunology and Phagocytosis: It enhances macrophage phagocytosis of apoptotic cells, aiding in tissue repair.
Metabolic Enzyme Studies: Deuterium-labeled compounds like RvD1-d5 are valuable tools for studying drug metabolism and pharmacokinetics.
Comparison with Similar Compounds
While RvD1-d5 is unique due to its deuterium labeling, it belongs to the broader family of resolvins derived from polyunsaturated fatty acids. Other related compounds include RvD1 (non-deuterated), RvD2, and RvD3.
Properties
IUPAC Name |
(4Z,7S,8R,9E,11E,13Z,15E,17S,19Z)-21,21,22,22,22-pentadeuterio-7,8,17-trihydroxydocosa-4,9,11,13,15,19-hexaenoic acid | |
---|---|---|
Details | Computed by Lexichem TK 2.7.0 (PubChem release 2021.08.13) | |
Source | PubChem | |
URL | https://pubchem.ncbi.nlm.nih.gov | |
Description | Data deposited in or computed by PubChem | |
InChI |
InChI=1S/C22H32O5/c1-2-3-9-14-19(23)15-10-6-4-5-7-11-16-20(24)21(25)17-12-8-13-18-22(26)27/h3-12,15-16,19-21,23-25H,2,13-14,17-18H2,1H3,(H,26,27)/b6-4-,7-5+,9-3-,12-8-,15-10+,16-11+/t19-,20+,21-/m0/s1/i1D3,2D2 | |
Details | Computed by InChI 1.0.6 (PubChem release 2021.08.13) | |
Source | PubChem | |
URL | https://pubchem.ncbi.nlm.nih.gov | |
Description | Data deposited in or computed by PubChem | |
InChI Key |
OIWTWACQMDFHJG-CLQVKFETSA-N | |
Details | Computed by InChI 1.0.6 (PubChem release 2021.08.13) | |
Source | PubChem | |
URL | https://pubchem.ncbi.nlm.nih.gov | |
Description | Data deposited in or computed by PubChem | |
Canonical SMILES |
CCC=CCC(C=CC=CC=CC=CC(C(CC=CCCC(=O)O)O)O)O | |
Details | Computed by OEChem 2.3.0 (PubChem release 2021.08.13) | |
Source | PubChem | |
URL | https://pubchem.ncbi.nlm.nih.gov | |
Description | Data deposited in or computed by PubChem | |
Isomeric SMILES |
[2H]C([2H])([2H])C([2H])([2H])/C=C\C[C@@H](\C=C\C=C/C=C/C=C/[C@H]([C@H](C/C=C\CCC(=O)O)O)O)O | |
Details | Computed by OEChem 2.3.0 (PubChem release 2021.08.13) | |
Source | PubChem | |
URL | https://pubchem.ncbi.nlm.nih.gov | |
Description | Data deposited in or computed by PubChem | |
Molecular Formula |
C22H32O5 | |
Details | Computed by PubChem 2.1 (PubChem release 2021.08.13) | |
Source | PubChem | |
URL | https://pubchem.ncbi.nlm.nih.gov | |
Description | Data deposited in or computed by PubChem | |
Molecular Weight |
381.5 g/mol | |
Details | Computed by PubChem 2.1 (PubChem release 2021.08.13) | |
Source | PubChem | |
URL | https://pubchem.ncbi.nlm.nih.gov | |
Description | Data deposited in or computed by PubChem | |
Retrosynthesis Analysis
AI-Powered Synthesis Planning: Our tool employs the Template_relevance Pistachio, Template_relevance Bkms_metabolic, Template_relevance Pistachio_ringbreaker, Template_relevance Reaxys, Template_relevance Reaxys_biocatalysis model, leveraging a vast database of chemical reactions to predict feasible synthetic routes.
One-Step Synthesis Focus: Specifically designed for one-step synthesis, it provides concise and direct routes for your target compounds, streamlining the synthesis process.
Accurate Predictions: Utilizing the extensive PISTACHIO, BKMS_METABOLIC, PISTACHIO_RINGBREAKER, REAXYS, REAXYS_BIOCATALYSIS database, our tool offers high-accuracy predictions, reflecting the latest in chemical research and data.
Strategy Settings
Precursor scoring | Relevance Heuristic |
---|---|
Min. plausibility | 0.01 |
Model | Template_relevance |
Template Set | Pistachio/Bkms_metabolic/Pistachio_ringbreaker/Reaxys/Reaxys_biocatalysis |
Top-N result to add to graph | 6 |
Feasible Synthetic Routes
Disclaimer and Information on In-Vitro Research Products
Please be aware that all articles and product information presented on BenchChem are intended solely for informational purposes. The products available for purchase on BenchChem are specifically designed for in-vitro studies, which are conducted outside of living organisms. In-vitro studies, derived from the Latin term "in glass," involve experiments performed in controlled laboratory settings using cells or tissues. It is important to note that these products are not categorized as medicines or drugs, and they have not received approval from the FDA for the prevention, treatment, or cure of any medical condition, ailment, or disease. We must emphasize that any form of bodily introduction of these products into humans or animals is strictly prohibited by law. It is essential to adhere to these guidelines to ensure compliance with legal and ethical standards in research and experimentation.