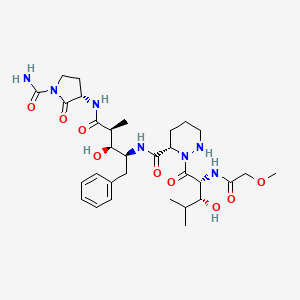
Padanamide A
Overview
Description
Padanamide A is a highly modified linear tetrapeptide notable for its absence of proteinogenic amino acids. It features two unusual heterocycles: (S)-3-amino-2-oxopyrrolidine-1-carboxamide and (S)-3-aminopiperidine-2,6-dione . This compound was discovered in marine sediment collected near Padana Nahua in Papua New Guinea and is produced by the marine actinomycete Streptomyces sp. RJA2928 .
Mechanism of Action
Target of Action
The primary targets of Padanamide A are believed to be involved in the biosynthesis of cysteine and methionine . These amino acids play crucial roles in various biological processes, including protein synthesis and metabolic pathways.
Mode of Action
This compound interacts with its targets by inhibiting the biosynthesis of cysteine and methionine . This inhibition disrupts the normal functioning of these amino acids, leading to changes in the cellular processes they are involved in.
Biochemical Pathways
The inhibition of cysteine and methionine biosynthesis by this compound affects several biochemical pathways. Cysteine and methionine are involved in protein synthesis and are precursors to other important molecules. For instance, cysteine is a precursor to glutathione, a powerful antioxidant, while methionine is essential for the synthesis of S-adenosylmethionine, a universal methyl donor .
Result of Action
The inhibition of cysteine and methionine biosynthesis by this compound leads to a disruption in the normal functioning of cells. This disruption can have various effects, depending on the specific roles of these amino acids in the cells . For instance, inhibition of cysteine biosynthesis can lead to a decrease in glutathione levels, potentially affecting the cell’s antioxidant capacity.
Action Environment
The action, efficacy, and stability of this compound can be influenced by various environmental factors. For instance, the pH and temperature of the environment can affect the stability and activity of the compound. Additionally, the presence of other molecules can influence the compound’s interaction with its targets .
Biochemical Analysis
Biochemical Properties
Padanamide A is synthesized by highly dissociated hybrid nonribosomal peptide synthetase/polyketide synthase machinery . It interacts with the carbamoyltransferase enzyme PadQ, which is critical to the formation of this compound . PadQ carbamoylates the rare biosynthetic precursor L-2,4-diaminobutyrate, generating L-2-amino-4-ureidobutyrate, the presumed precursor to the C-terminal residue of this compound .
Cellular Effects
A chemical genomics analysis using yeast deletion mutants suggests that this compound is involved in the inhibition of cysteine and methionine biosynthesis . These amino acids are involved in the yeast’s response to the peptide .
Molecular Mechanism
The molecular mechanism of this compound involves the carbamoylation of L-2,4-diaminobutyrate by the enzyme PadQ . This process generates L-2-amino-4-ureidobutyrate, which is the presumed precursor to the C-terminal residue of this compound .
Metabolic Pathways
The compound is known to interact with the enzyme PadQ in the process of carbamoylation . This could potentially affect metabolic flux or metabolite levels.
Preparation Methods
Synthetic Routes and Reaction Conditions: Padanamide A is synthesized by a hybrid nonribosomal peptide synthetase/polyketide synthase machinery . The biosynthetic pathway involves the carbamoylation of L-2,4-diaminobutyrate by the enzyme PadQ, generating L-2-amino-4-ureidobutyrate, which is the precursor to the C-terminal residue of this compound . An unusual thioesterase-catalyzed cyclization is proposed to generate the heterocycles present in the compound .
Industrial Production Methods: Currently, this compound is produced in laboratory cultures of Streptomyces sp. isolated from marine sediment . The industrial production methods are still under research and development, focusing on optimizing the yield and purity of the compound.
Chemical Reactions Analysis
Types of Reactions: Padanamide A undergoes various chemical reactions, including hydrolysis and derivatization .
Common Reagents and Conditions:
Hydrolysis: Acid hydrolysis of this compound followed by derivatization with 1-fluoro-2,4-dinitrobenzene.
Derivatization: Derivatization of amino acid standards with Marfey’s reagent.
Major Products: The major products formed from these reactions include derivatized amino acids, which are analyzed using high-performance liquid chromatography (HPLC) .
Scientific Research Applications
Padanamide A has several scientific research applications:
Comparison with Similar Compounds
Padanamide B: Similar to Padanamide A but features a different C-terminal residue derived from glutamine.
Actinoramide A: Identical to this compound but isolated from a different Streptomyces strain.
Uniqueness: this compound is unique due to its highly modified linear tetrapeptide structure and the absence of proteinogenic amino acids . The presence of unusual heterocycles and its biosynthetic pathway involving hybrid nonribosomal peptide synthetase/polyketide synthase machinery further distinguishes it from other compounds .
Properties
IUPAC Name |
(3S)-N-[(2S,3S,4S)-5-[[(3S)-1-carbamoyl-2-oxopyrrolidin-3-yl]amino]-3-hydroxy-4-methyl-5-oxo-1-phenylpentan-2-yl]-2-[(2R,3R)-3-hydroxy-2-[(2-methoxyacetyl)amino]-4-methylpentanoyl]diazinane-3-carboxamide | |
---|---|---|
Details | Computed by Lexichem TK 2.7.0 (PubChem release 2021.05.07) | |
Source | PubChem | |
URL | https://pubchem.ncbi.nlm.nih.gov | |
Description | Data deposited in or computed by PubChem | |
InChI |
InChI=1S/C31H47N7O9/c1-17(2)25(40)24(36-23(39)16-47-4)30(45)38-22(11-8-13-33-38)28(43)35-21(15-19-9-6-5-7-10-19)26(41)18(3)27(42)34-20-12-14-37(29(20)44)31(32)46/h5-7,9-10,17-18,20-22,24-26,33,40-41H,8,11-16H2,1-4H3,(H2,32,46)(H,34,42)(H,35,43)(H,36,39)/t18-,20-,21-,22-,24+,25+,26-/m0/s1 | |
Details | Computed by InChI 1.0.6 (PubChem release 2021.05.07) | |
Source | PubChem | |
URL | https://pubchem.ncbi.nlm.nih.gov | |
Description | Data deposited in or computed by PubChem | |
InChI Key |
IAYPOIKGUHHBAU-DYTCPEOESA-N | |
Details | Computed by InChI 1.0.6 (PubChem release 2021.05.07) | |
Source | PubChem | |
URL | https://pubchem.ncbi.nlm.nih.gov | |
Description | Data deposited in or computed by PubChem | |
Canonical SMILES |
CC(C)C(C(C(=O)N1C(CCCN1)C(=O)NC(CC2=CC=CC=C2)C(C(C)C(=O)NC3CCN(C3=O)C(=O)N)O)NC(=O)COC)O | |
Details | Computed by OEChem 2.3.0 (PubChem release 2021.05.07) | |
Source | PubChem | |
URL | https://pubchem.ncbi.nlm.nih.gov | |
Description | Data deposited in or computed by PubChem | |
Isomeric SMILES |
C[C@@H]([C@@H]([C@H](CC1=CC=CC=C1)NC(=O)[C@@H]2CCCNN2C(=O)[C@@H]([C@@H](C(C)C)O)NC(=O)COC)O)C(=O)N[C@H]3CCN(C3=O)C(=O)N | |
Details | Computed by OEChem 2.3.0 (PubChem release 2021.05.07) | |
Source | PubChem | |
URL | https://pubchem.ncbi.nlm.nih.gov | |
Description | Data deposited in or computed by PubChem | |
Molecular Formula |
C31H47N7O9 | |
Details | Computed by PubChem 2.1 (PubChem release 2021.05.07) | |
Source | PubChem | |
URL | https://pubchem.ncbi.nlm.nih.gov | |
Description | Data deposited in or computed by PubChem | |
Molecular Weight |
661.7 g/mol | |
Details | Computed by PubChem 2.1 (PubChem release 2021.05.07) | |
Source | PubChem | |
URL | https://pubchem.ncbi.nlm.nih.gov | |
Description | Data deposited in or computed by PubChem | |
Retrosynthesis Analysis
AI-Powered Synthesis Planning: Our tool employs the Template_relevance Pistachio, Template_relevance Bkms_metabolic, Template_relevance Pistachio_ringbreaker, Template_relevance Reaxys, Template_relevance Reaxys_biocatalysis model, leveraging a vast database of chemical reactions to predict feasible synthetic routes.
One-Step Synthesis Focus: Specifically designed for one-step synthesis, it provides concise and direct routes for your target compounds, streamlining the synthesis process.
Accurate Predictions: Utilizing the extensive PISTACHIO, BKMS_METABOLIC, PISTACHIO_RINGBREAKER, REAXYS, REAXYS_BIOCATALYSIS database, our tool offers high-accuracy predictions, reflecting the latest in chemical research and data.
Strategy Settings
Precursor scoring | Relevance Heuristic |
---|---|
Min. plausibility | 0.01 |
Model | Template_relevance |
Template Set | Pistachio/Bkms_metabolic/Pistachio_ringbreaker/Reaxys/Reaxys_biocatalysis |
Top-N result to add to graph | 6 |
Feasible Synthetic Routes
Disclaimer and Information on In-Vitro Research Products
Please be aware that all articles and product information presented on BenchChem are intended solely for informational purposes. The products available for purchase on BenchChem are specifically designed for in-vitro studies, which are conducted outside of living organisms. In-vitro studies, derived from the Latin term "in glass," involve experiments performed in controlled laboratory settings using cells or tissues. It is important to note that these products are not categorized as medicines or drugs, and they have not received approval from the FDA for the prevention, treatment, or cure of any medical condition, ailment, or disease. We must emphasize that any form of bodily introduction of these products into humans or animals is strictly prohibited by law. It is essential to adhere to these guidelines to ensure compliance with legal and ethical standards in research and experimentation.