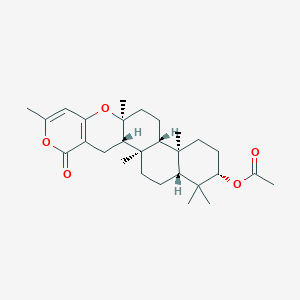
Chevalone B
Overview
Description
Chevalone B is a meroterpenoid compound isolated from the ethyl acetate extract of the marine sponge-associated fungus Aspergillus similanensis. It has shown significant antimicrobial activity against Gram-positive and Gram-negative bacteria, Candida albicans, and multidrug-resistant strains from the environment . The structure of this compound was confirmed by 1D and 2D NMR spectroscopy .
Preparation Methods
Synthetic Routes and Reaction Conditions: Chevalone B is typically isolated from natural sources rather than synthesized chemically. The primary source is the marine sponge-associated fungus Aspergillus similanensis. The extraction process involves using ethyl acetate to obtain the compound from the fungal culture .
Industrial Production Methods: Currently, there are no large-scale industrial production methods for this compound. The compound is mainly produced in research laboratories through the extraction from fungal cultures .
Chemical Reactions Analysis
Types of Reactions: Chevalone B undergoes various chemical reactions, including oxidation, reduction, and substitution reactions.
Common Reagents and Conditions:
Oxidation: Common oxidizing agents such as potassium permanganate or chromium trioxide can be used.
Reduction: Reducing agents like sodium borohydride or lithium aluminum hydride are typically employed.
Substitution: Various nucleophiles can be used under acidic or basic conditions to introduce different functional groups.
Major Products: The major products formed from these reactions depend on the specific reagents and conditions used. For example, oxidation can lead to the formation of ketones or carboxylic acids, while reduction can yield alcohols .
Scientific Research Applications
Antimicrobial Activity
Chevalone B exhibits notable antimicrobial properties, albeit with relatively weak efficacy compared to other compounds in its class.
- Activity Against Bacteria : In studies, this compound demonstrated minimal antibacterial activity against Staphylococcus aureus, with a minimum inhibitory concentration (MIC) of 50 µg/mL . This suggests potential but limited use as an antibacterial agent.
- Synergistic Effects : While this compound alone shows weak antibacterial properties, its potential synergistic effects with other antibiotics have been noted. For example, it may enhance the activity of certain antibiotics against resistant strains .
Cytotoxicity and Anticancer Properties
This compound has been investigated for its cytotoxic effects on various cancer cell lines:
- Cytotoxic Activity : The compound has shown significant cytotoxicity against KB (human oral epidermoid carcinoma) and NCI-H187 (human small cell lung cancer) cells, with IC50 values of 2.9 µg/mL and 9.8 µg/mL, respectively . This indicates a strong potential for use in cancer therapeutics.
- Combination Therapies : Research has indicated that this compound can be effective when used in combination with other chemotherapeutic agents. For instance, it has been observed to enhance the cytotoxic effects of doxorubicin on breast cancer cells .
Structure-Activity Relationship Studies
Understanding the structure-activity relationship (SAR) of this compound is crucial for developing more potent derivatives:
- Chemical Derivatization : Recent studies have focused on chemically modifying this compound to create analogues with improved biological activities. These modifications aim to enhance its efficacy against cancer cells and bacteria while reducing toxicity .
- Crystallographic Studies : The crystallographic data and absolute stereochemistry of this compound have been determined, providing insights into its molecular structure that can inform future modifications and applications .
Case Studies
Several case studies highlight the applications of this compound in research:
- Study on Antimicrobial Compounds : A comprehensive study involving various marine-derived fungi reported on the antimicrobial properties of this compound alongside other meroterpenoids. The findings suggest that while this compound exhibits weaker activity, it contributes to a broader spectrum of antimicrobial agents derived from marine sources .
- Research on Cancer Cell Lines : In vitro studies have demonstrated that this compound effectively inhibits cell viability in multiple cancer cell lines, suggesting its potential as a lead compound for further development in anticancer therapies .
Mechanism of Action
Chevalone B exerts its antimicrobial effects by disrupting the cell membrane integrity of bacteria and fungi. This disruption leads to cell lysis and death. The exact molecular targets and pathways involved are still under investigation, but it is believed that this compound interacts with membrane lipids and proteins .
Comparison with Similar Compounds
Chevalone B is part of a larger family of meroterpenoids, which include compounds like Chevalone A, Chevalone C, and Chevalone E . These compounds share similar structural features but differ in their specific functional groups and biological activities. For example:
Chevalone A: Known for its antimalarial activity.
Chevalone C: Exhibits antimycobacterial activity.
Chevalone E: Enhances the antibiotic effect of oxacillin against methicillin-resistant Staphylococcus aureus
Biological Activity
Chevalone B is a meroterpenoid compound derived from the fungus Eurotium chevalieri, notable for its diverse biological activities. This article presents a comprehensive overview of the biological activity of this compound, including its cytotoxic effects, antimicrobial properties, and potential therapeutic applications.
Chemical Structure and Derivation
This compound is part of a larger family of chevalones, which are characterized by their complex structures. The compound has been isolated from various fungal sources, particularly marine-derived fungi. Its structural features contribute to its biological activities, making it a subject of interest in pharmacological research.
1. Cytotoxic Effects
This compound exhibits significant cytotoxicity against various cancer cell lines. Research indicates that it has IC50 values of:
Cell Line | IC50 (µg/ml) |
---|---|
Lung carcinoma cells | 3.9 |
Epidermal carcinoma cells | 2.9 |
Breast cancer cell line (MDA-MB-231) | Synergistic with doxorubicin |
These findings suggest that this compound may enhance the efficacy of existing chemotherapeutic agents, potentially leading to improved treatment outcomes for cancer patients .
2. Antimicrobial Activity
This compound has demonstrated antimicrobial properties, particularly against antibiotic-resistant strains. Notably, Chevalone E, a related compound, has shown synergistic effects with oxacillin against methicillin-resistant Staphylococcus aureus (MRSA) . This synergy highlights the potential for this compound and its analogs in combating resistant bacterial infections.
The mechanisms underlying the biological activities of this compound are still under investigation. However, studies suggest that its cytotoxic effects may be mediated through apoptosis induction in cancer cells and disruption of bacterial cell wall synthesis in pathogens. Further research is necessary to elucidate these mechanisms fully.
Case Studies and Research Findings
Several studies have explored the biological activity of this compound and its derivatives:
- Synergistic Effects : A study found that this compound enhances the cytotoxic effect of doxorubicin in breast cancer cells, indicating a potential for combination therapies in oncology .
- Antimicrobial Synergy : Research demonstrated that Chevalone E can enhance the activity of antibiotics against resistant strains, suggesting that this compound may share similar properties .
Properties
IUPAC Name |
[(1R,2S,11S,14R,15R,18S,20R)-1,7,11,15,19,19-hexamethyl-5-oxo-6,10-dioxapentacyclo[12.8.0.02,11.04,9.015,20]docosa-4(9),7-dien-18-yl] acetate | |
---|---|---|
Details | Computed by LexiChem 2.6.6 (PubChem release 2019.06.18) | |
Source | PubChem | |
URL | https://pubchem.ncbi.nlm.nih.gov | |
Description | Data deposited in or computed by PubChem | |
InChI |
InChI=1S/C28H40O5/c1-16-14-19-18(24(30)31-16)15-22-27(6)11-8-20-25(3,4)23(32-17(2)29)10-12-26(20,5)21(27)9-13-28(22,7)33-19/h14,20-23H,8-13,15H2,1-7H3/t20-,21+,22-,23-,26-,27+,28-/m0/s1 | |
Details | Computed by InChI 1.0.5 (PubChem release 2019.06.18) | |
Source | PubChem | |
URL | https://pubchem.ncbi.nlm.nih.gov | |
Description | Data deposited in or computed by PubChem | |
InChI Key |
IRSLQXSSQAASGV-GPTGPEQGSA-N | |
Details | Computed by InChI 1.0.5 (PubChem release 2019.06.18) | |
Source | PubChem | |
URL | https://pubchem.ncbi.nlm.nih.gov | |
Description | Data deposited in or computed by PubChem | |
Canonical SMILES |
CC1=CC2=C(CC3C4(CCC5C(C(CCC5(C4CCC3(O2)C)C)OC(=O)C)(C)C)C)C(=O)O1 | |
Details | Computed by OEChem 2.1.5 (PubChem release 2019.06.18) | |
Source | PubChem | |
URL | https://pubchem.ncbi.nlm.nih.gov | |
Description | Data deposited in or computed by PubChem | |
Isomeric SMILES |
CC1=CC2=C(C[C@H]3[C@@]4(CC[C@@H]5[C@@]([C@H]4CC[C@@]3(O2)C)(CC[C@@H](C5(C)C)OC(=O)C)C)C)C(=O)O1 | |
Details | Computed by OEChem 2.1.5 (PubChem release 2019.06.18) | |
Source | PubChem | |
URL | https://pubchem.ncbi.nlm.nih.gov | |
Description | Data deposited in or computed by PubChem | |
Molecular Formula |
C28H40O5 | |
Details | Computed by PubChem 2.1 (PubChem release 2019.06.18) | |
Source | PubChem | |
URL | https://pubchem.ncbi.nlm.nih.gov | |
Description | Data deposited in or computed by PubChem | |
Molecular Weight |
456.6 g/mol | |
Details | Computed by PubChem 2.1 (PubChem release 2021.05.07) | |
Source | PubChem | |
URL | https://pubchem.ncbi.nlm.nih.gov | |
Description | Data deposited in or computed by PubChem | |
Retrosynthesis Analysis
AI-Powered Synthesis Planning: Our tool employs the Template_relevance Pistachio, Template_relevance Bkms_metabolic, Template_relevance Pistachio_ringbreaker, Template_relevance Reaxys, Template_relevance Reaxys_biocatalysis model, leveraging a vast database of chemical reactions to predict feasible synthetic routes.
One-Step Synthesis Focus: Specifically designed for one-step synthesis, it provides concise and direct routes for your target compounds, streamlining the synthesis process.
Accurate Predictions: Utilizing the extensive PISTACHIO, BKMS_METABOLIC, PISTACHIO_RINGBREAKER, REAXYS, REAXYS_BIOCATALYSIS database, our tool offers high-accuracy predictions, reflecting the latest in chemical research and data.
Strategy Settings
Precursor scoring | Relevance Heuristic |
---|---|
Min. plausibility | 0.01 |
Model | Template_relevance |
Template Set | Pistachio/Bkms_metabolic/Pistachio_ringbreaker/Reaxys/Reaxys_biocatalysis |
Top-N result to add to graph | 6 |
Feasible Synthetic Routes
Q1: What is the origin of Chevalone B and what other compounds are often found alongside it?
A1: this compound is a secondary metabolite produced by various fungal species, including Aspergillus sp., Xylaria humosa, and Neosartorya species. It is often co-isolated with other meroterpenoids like Chevalone C and sartorypyrones, as well as various indole alkaloids like tryptoquivalines. [, , , ]
Q2: Does this compound exhibit any biological activity?
A2: While some studies indicate weak antibacterial activity, this compound generally demonstrates limited bioactivity in standard antimicrobial assays. For instance, it showed weak activity against Staphylococcus aureus and Enterococcus faecalis. [, , ] More research is needed to fully explore its potential bioactivities.
Q3: What is known about the structure of this compound?
A3: While the exact molecular formula and weight are not specified in the provided abstracts, the research confirms that this compound is a meroterpenoid, indicating it possesses a structure partially derived from terpenoid precursors. Its absolute stereochemistry has been determined through X-ray crystallography. []
Q4: Has the structure of this compound been modified to investigate structure-activity relationships (SAR)?
A4: While the provided research doesn't explicitly detail SAR studies on this compound, one study describes the isolation of Chevalone E, a new derivative, from the fungus Aspergillus similanensis. [] This discovery could pave the way for future investigations into the impact of structural modifications on this compound's activity.
Q5: What analytical techniques are used to characterize and study this compound?
A5: Researchers utilize a combination of techniques for this compound characterization, including:
- Nuclear Magnetic Resonance (NMR) spectroscopy: This provides crucial structural information by analyzing the magnetic properties of atomic nuclei. []
- X-ray crystallography: This technique determines the three-dimensional structure of molecules, offering insights into the absolute configuration of this compound. [, ]
- High-performance liquid chromatography (HPLC): This method is used to separate, identify, and quantify components in a mixture. []
Disclaimer and Information on In-Vitro Research Products
Please be aware that all articles and product information presented on BenchChem are intended solely for informational purposes. The products available for purchase on BenchChem are specifically designed for in-vitro studies, which are conducted outside of living organisms. In-vitro studies, derived from the Latin term "in glass," involve experiments performed in controlled laboratory settings using cells or tissues. It is important to note that these products are not categorized as medicines or drugs, and they have not received approval from the FDA for the prevention, treatment, or cure of any medical condition, ailment, or disease. We must emphasize that any form of bodily introduction of these products into humans or animals is strictly prohibited by law. It is essential to adhere to these guidelines to ensure compliance with legal and ethical standards in research and experimentation.