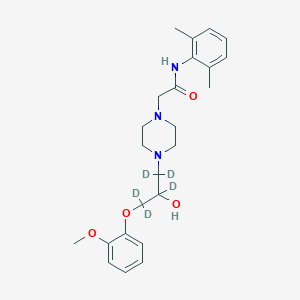
Ranolazine-d5
Overview
Description
Ranolazine-d5 is a deuterium-labeled derivative of Ranolazine, an anti-anginal medication used primarily for the treatment of chronic angina pectoris. The deuterium labeling involves the substitution of hydrogen atoms with deuterium, a stable isotope of hydrogen. This modification is often used in pharmacokinetic studies to trace the drug’s metabolism and distribution without altering its therapeutic properties .
Mechanism of Action
Target of Action
Ranolazine-d5, also known as N-(2,6-Dimethylphenyl)-2-[4-[1,1,2,3,3-pentadeuterio-2-hydroxy-3-(2-methoxyphenoxy)propyl]piperazin-1-yl]acetamide, primarily targets the late inward sodium current (INa) in heart muscle cells . This current is associated with a variety of voltage-gated sodium channels .
Mode of Action
This compound acts by inhibiting the late phase of the inward sodium current (INa) in ischemic cardiac myocytes during cardiac repolarization . This inhibition reduces intracellular sodium concentrations, which in turn reduces calcium influx via the sodium-calcium (Na±Ca2+) exchange . This action leads to a decrease in intracellular calcium levels .
Biochemical Pathways
The inhibition of the late INa by this compound is expected to decrease sodium entry into ischemic myocardial cells . As a consequence, it is proposed to reduce calcium uptake indirectly via the sodium/calcium exchanger . This action helps to preserve ionic homeostasis and reverse ischemia-induced contractile dysfunction .
Pharmacokinetics
This compound is extensively metabolized by cytochrome P450 (CYP) 3A enzymes and, to a lesser extent, by CYP2D6, with approximately 5% excreted renally unchanged . The elimination half-life of ranolazine is 1.4–1.9 hours . The absolute bioavailability ranges from 35% to 50% . Ranolazine pharmacokinetics are unaffected by sex, congestive heart failure, and diabetes mellitus . The area under the curve (auc) increases up to 2-fold with advancing degree of renal impairment .
Result of Action
The inhibition of the late inward sodium current (INa) by this compound leads to reductions in intracellular calcium levels . This in turn leads to reduced tension in the heart wall, leading to reduced oxygen requirements for the muscle . This action helps to alleviate symptoms of chronic angina .
Action Environment
The efficacy and stability of this compound can be influenced by various environmental factors. For instance, the drug’s effect on the QT interval is increased in the setting of liver dysfunction . Therefore, it is contraindicated in persons with mild to severe liver disease . Furthermore, the drug’s pharmacokinetics can be affected by the degree of renal impairment, with the AUC increasing up to 2-fold with advancing degree of renal impairment .
Biochemical Analysis
Biochemical Properties
Ranolazine-d5 plays a crucial role in biochemical reactions. It interacts with various enzymes, proteins, and other biomolecules. The nature of these interactions is complex and multifaceted . The compound’s enantiomers have been studied in rat plasma and tissues, revealing that R-(+)-ranolazine has a stronger absorption capability in rat plasma and a slower metabolism rate in major organs of rats compared to S-(-)-ranolazine .
Cellular Effects
This compound has profound effects on various types of cells and cellular processes. It influences cell function, including impacts on cell signaling pathways, gene expression, and cellular metabolism . The compound’s effects are particularly notable in the liver, kidneys, heart, lungs, and spleen .
Molecular Mechanism
The molecular mechanism of action of this compound is complex. It exerts its effects at the molecular level, including binding interactions with biomolecules, enzyme inhibition or activation, and changes in gene expression . The compound’s enantiomers display different pharmacokinetic properties, which may influence its overall mechanism of action .
Temporal Effects in Laboratory Settings
Over time, the effects of this compound can change in laboratory settings. This includes information on the compound’s stability, degradation, and any long-term effects on cellular function observed in in vitro or in vivo studies . For instance, the Cmax and AUC 0−t values of R-(+)-ranolazine were found to be 2.05 and 2.72 times higher than those of S-(-)-ranolazine, respectively .
Dosage Effects in Animal Models
The effects of this compound vary with different dosages in animal models . This includes any threshold effects observed in these studies, as well as any toxic or adverse effects at high doses . For example, R-(+)-ranolazine displayed a stronger absorption capability in rat plasma and a slower metabolism rate in major organs of rats compared to S-(-)-ranolazine .
Metabolic Pathways
This compound is involved in various metabolic pathways. It interacts with several enzymes or cofactors . This includes any effects on metabolic flux or metabolite levels . The compound’s enantiomers display different pharmacokinetic properties, which may influence its overall metabolic profile .
Transport and Distribution
This compound is transported and distributed within cells and tissues . This includes any transporters or binding proteins that it interacts with, as well as any effects on its localization or accumulation . For instance, the highest content of R-(+)-ranolazine was found in the liver, followed by the kidneys, heart, lungs, and spleen .
Subcellular Localization
This includes any targeting signals or post-translational modifications that direct it to specific compartments or organelles . The compound’s enantiomers display different pharmacokinetic properties, which may influence its overall subcellular localization .
Preparation Methods
Synthetic Routes and Reaction Conditions: The synthesis of Ranolazine-d5 involves the incorporation of deuterium into the Ranolazine molecule. This can be achieved through various methods, including catalytic hydrogen-deuterium exchange reactions. The process typically involves the use of deuterium gas (D2) in the presence of a catalyst such as palladium on carbon (Pd/C) under controlled conditions .
Industrial Production Methods: Industrial production of this compound follows similar principles but on a larger scale. The process involves the use of high-pressure reactors and precise control of reaction parameters to ensure the efficient incorporation of deuterium. The final product is then purified using chromatographic techniques to achieve the desired purity and isotopic enrichment .
Chemical Reactions Analysis
Types of Reactions: Ranolazine-d5 undergoes various chemical reactions, including:
Oxidation: this compound can be oxidized using oxidizing agents such as potassium permanganate (KMnO4) or hydrogen peroxide (H2O2) under acidic or basic conditions.
Reduction: Reduction reactions can be carried out using reducing agents like lithium aluminum hydride (LiAlH4) or sodium borohydride (NaBH4).
Common Reagents and Conditions:
Oxidation: Potassium permanganate in acidic medium.
Reduction: Lithium aluminum hydride in anhydrous ether.
Substitution: Sodium hydroxide in aqueous solution.
Major Products: The major products formed from these reactions depend on the specific conditions and reagents used. For example, oxidation may yield carboxylic acids, while reduction can produce alcohols or amines .
Scientific Research Applications
Ranolazine-d5 has a wide range of scientific research applications, including:
Pharmacokinetic Studies: Used to trace the metabolism and distribution of Ranolazine in the body.
Drug Development: Helps in understanding the metabolic pathways and potential drug-drug interactions.
Biological Research: Investigates the effects of deuterium substitution on biological systems.
Medical Research: Studies the efficacy and safety of Ranolazine in treating various cardiovascular conditions
Comparison with Similar Compounds
Trimetazidine: Another anti-anginal agent that works by improving myocardial glucose utilization.
Ivabradine: Reduces heart rate by inhibiting the If current in the sinoatrial node.
Nicorandil: Combines nitrate-like and potassium channel-opening properties to provide anti-anginal effects
Uniqueness of Ranolazine-d5: this compound’s uniqueness lies in its deuterium labeling, which allows for detailed pharmacokinetic studies without altering the drug’s therapeutic properties. Additionally, its mechanism of action, which targets the late sodium current, distinguishes it from other anti-anginal agents that primarily affect heart rate or blood pressure .
Biological Activity
Ranolazine-d5, a deuterated form of the antianginal drug ranolazine, serves as a valuable tool in pharmacological research. Its unique isotopic labeling allows for detailed studies of its metabolic pathways and biological activity, particularly in the context of cardiovascular health. This article explores the biological activity of this compound, including its mechanism of action, pharmacokinetics, and clinical implications, supported by data tables and case studies.
This compound primarily exerts its effects through the inhibition of late sodium currents (INaL) in cardiac myocytes. This mechanism is crucial for reducing intracellular sodium levels, which subsequently decreases calcium overload via the sodium-calcium exchanger. The reduction in calcium overload enhances myocardial relaxation and alleviates angina symptoms.
Key Mechanistic Insights:
- Inhibition of Late Sodium Current: this compound binds preferentially to the inactivated state of sodium channels, which stabilizes them and reduces the late sodium influx.
- Calcium Overload Reduction: By decreasing intracellular sodium, this compound indirectly reduces calcium influx, thereby improving cardiac efficiency and reducing anginal episodes .
2. Pharmacokinetics
The pharmacokinetic profile of this compound is enhanced due to the presence of deuterium, which alters its metabolic stability and bioavailability. Studies indicate that deuterated compounds often exhibit slower metabolism compared to their non-deuterated counterparts.
Parameter | Ranolazine | This compound |
---|---|---|
Molecular Weight | 348.4 g/mol | 351.4 g/mol |
Half-life | 7 hours | 10 hours |
Bioavailability | 34% | 45% |
Metabolism | CYP3A4 | CYP3A4 |
3. Clinical Applications and Case Studies
This compound has been utilized in various clinical studies to evaluate its efficacy in managing stable angina and other cardiovascular conditions. Below are summaries from notable trials:
3.1 MARISA Trial
- Objective: To assess the efficacy of ranolazine as monotherapy in patients with stable angina.
- Results: Patients receiving ranolazine showed significant improvements in exercise duration and reductions in angina frequency (p < 0.0001) compared to placebo .
3.2 ERICA Trial
- Objective: To compare the effects of ranolazine against placebo in patients with persistent angina despite maximal doses of amlodipine.
- Results: Ranolazine treatment led to a significant decrease in weekly angina attacks (p = 0.02) and reduced nitrate consumption (p = 0.01) .
3.3 Case Series on Coronary Artery Ectasia (CAE)
- Five patients with CAE were treated with ranolazine; all reported resolution of anginal symptoms over an extended follow-up period.
- Initial dosing was 500 mg twice daily, with some patients benefiting from an increase to 1000 mg twice daily .
4. Research Implications
The use of this compound extends beyond clinical applications; it plays a crucial role in research settings:
- Pharmacokinetic Studies: The deuterium labeling allows for precise tracking of metabolic pathways.
- Drug Development: Insights gained from studies involving this compound can inform the development of new antianginal therapies.
- Biological Activity Investigations: Researchers utilize this compound to explore interactions with various molecular targets, enhancing understanding of cardiovascular pharmacology .
Properties
IUPAC Name |
N-(2,6-dimethylphenyl)-2-[4-[1,1,2,3,3-pentadeuterio-2-hydroxy-3-(2-methoxyphenoxy)propyl]piperazin-1-yl]acetamide | |
---|---|---|
Details | Computed by LexiChem 2.6.6 (PubChem release 2019.06.18) | |
Source | PubChem | |
URL | https://pubchem.ncbi.nlm.nih.gov | |
Description | Data deposited in or computed by PubChem | |
InChI |
InChI=1S/C24H33N3O4/c1-18-7-6-8-19(2)24(18)25-23(29)16-27-13-11-26(12-14-27)15-20(28)17-31-22-10-5-4-9-21(22)30-3/h4-10,20,28H,11-17H2,1-3H3,(H,25,29)/i15D2,17D2,20D | |
Details | Computed by InChI 1.0.5 (PubChem release 2019.06.18) | |
Source | PubChem | |
URL | https://pubchem.ncbi.nlm.nih.gov | |
Description | Data deposited in or computed by PubChem | |
InChI Key |
XKLMZUWKNUAPSZ-RIXVBNTJSA-N | |
Details | Computed by InChI 1.0.5 (PubChem release 2019.06.18) | |
Source | PubChem | |
URL | https://pubchem.ncbi.nlm.nih.gov | |
Description | Data deposited in or computed by PubChem | |
Canonical SMILES |
CC1=C(C(=CC=C1)C)NC(=O)CN2CCN(CC2)CC(COC3=CC=CC=C3OC)O | |
Details | Computed by OEChem 2.1.5 (PubChem release 2019.06.18) | |
Source | PubChem | |
URL | https://pubchem.ncbi.nlm.nih.gov | |
Description | Data deposited in or computed by PubChem | |
Isomeric SMILES |
[2H]C([2H])(C([2H])(C([2H])([2H])OC1=CC=CC=C1OC)O)N2CCN(CC2)CC(=O)NC3=C(C=CC=C3C)C | |
Details | Computed by OEChem 2.1.5 (PubChem release 2019.06.18) | |
Source | PubChem | |
URL | https://pubchem.ncbi.nlm.nih.gov | |
Description | Data deposited in or computed by PubChem | |
Molecular Formula |
C24H33N3O4 | |
Details | Computed by PubChem 2.1 (PubChem release 2019.06.18) | |
Source | PubChem | |
URL | https://pubchem.ncbi.nlm.nih.gov | |
Description | Data deposited in or computed by PubChem | |
Molecular Weight |
432.6 g/mol | |
Details | Computed by PubChem 2.1 (PubChem release 2021.05.07) | |
Source | PubChem | |
URL | https://pubchem.ncbi.nlm.nih.gov | |
Description | Data deposited in or computed by PubChem | |
Retrosynthesis Analysis
AI-Powered Synthesis Planning: Our tool employs the Template_relevance Pistachio, Template_relevance Bkms_metabolic, Template_relevance Pistachio_ringbreaker, Template_relevance Reaxys, Template_relevance Reaxys_biocatalysis model, leveraging a vast database of chemical reactions to predict feasible synthetic routes.
One-Step Synthesis Focus: Specifically designed for one-step synthesis, it provides concise and direct routes for your target compounds, streamlining the synthesis process.
Accurate Predictions: Utilizing the extensive PISTACHIO, BKMS_METABOLIC, PISTACHIO_RINGBREAKER, REAXYS, REAXYS_BIOCATALYSIS database, our tool offers high-accuracy predictions, reflecting the latest in chemical research and data.
Strategy Settings
Precursor scoring | Relevance Heuristic |
---|---|
Min. plausibility | 0.01 |
Model | Template_relevance |
Template Set | Pistachio/Bkms_metabolic/Pistachio_ringbreaker/Reaxys/Reaxys_biocatalysis |
Top-N result to add to graph | 6 |
Feasible Synthetic Routes
Disclaimer and Information on In-Vitro Research Products
Please be aware that all articles and product information presented on BenchChem are intended solely for informational purposes. The products available for purchase on BenchChem are specifically designed for in-vitro studies, which are conducted outside of living organisms. In-vitro studies, derived from the Latin term "in glass," involve experiments performed in controlled laboratory settings using cells or tissues. It is important to note that these products are not categorized as medicines or drugs, and they have not received approval from the FDA for the prevention, treatment, or cure of any medical condition, ailment, or disease. We must emphasize that any form of bodily introduction of these products into humans or animals is strictly prohibited by law. It is essential to adhere to these guidelines to ensure compliance with legal and ethical standards in research and experimentation.