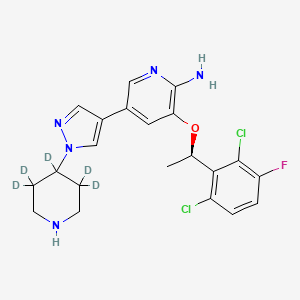
Crizotinib-d5
Overview
Description
(R)-Crizotinib-d5 is intended for use as an internal standard for the quantification of (R)-crizotinib by GC- or LC-MS. (R)-Crizotinib is a derivative of aminopyridine that acts as a potent, orally bioavailable, ATP-competitive small-molecule dual inhibitor of c-MET (IC50 = 8 nM) and ALK (IC50 = 20 nM) receptor tyrosine kinases. (R)-Crizotinib shows antitumor efficacy, including cytoreductive antitumor activity, in multiple tumor models implanted in athymic mice that express activated c-MET or ALK fusion proteins (IC50s = 5-20 nM).
Mechanism of Action
Target of Action
Crizotinib-d5, also known as 3-[(1R)-1-(2,6-Dichloro-3-fluorophenyl)ethoxy]-5-[1-(3,3,4,5,5-pentadeuteriopiperidin-4-yl)pyrazol-4-yl]pyridin-2-amine, is a potent inhibitor of several tyrosine kinases. Its primary targets include anaplastic lymphoma kinase (ALK), hepatocyte growth factor receptor (HGFR, c-MET), ROS1 (c-ros), and Recepteur d’Origine Nantais (RON) tyrosine kinases . These kinases play crucial roles in cell proliferation, survival, and differentiation, and their dysregulation is often associated with the development of various cancers .
Mode of Action
this compound acts by competitively binding to the ATP-binding pocket of its target kinases, thereby inhibiting their activity . This inhibition disrupts the signaling pathways regulated by these kinases, leading to reduced cell proliferation and increased apoptosis . For instance, in the case of ALK, this compound can inhibit the kinase activity of the EML4-ALK fusion protein, which is often present in non-small cell lung cancer (NSCLC) patients .
Biochemical Pathways
The inhibition of ALK, HGFR, ROS1, and RON by this compound affects several biochemical pathways. For example, the drug’s action on ALK can disrupt the downstream PI3K/AKT/mTOR and RAS/RAF/MEK/ERK pathways, which are involved in cell survival and proliferation . Similarly, the inhibition of HGFR can affect the PI3K/AKT pathway and the RAS/RAF/MEK/ERK pathway, both of which play roles in cell growth and survival .
Pharmacokinetics
this compound is orally bioavailable, with a median time to maximal plasma concentration (Tmax) of approximately 4 hours and a mean apparent terminal half-life of 42 hours in patients with cancer after a single 250-mg oral dose . The drug is metabolized primarily by the liver, specifically by the CYP3A4/3A5 enzymes . Its pharmacokinetics can be influenced by factors such as body weight, creatinine clearance, and total bilirubin .
Result of Action
The molecular and cellular effects of this compound’s action include the inhibition of cell proliferation, induction of apoptosis, and reduction of tumor growth . By inhibiting the activity of its target kinases, this compound disrupts the signaling pathways that promote cell survival and proliferation, leading to cell death .
Action Environment
Environmental factors can influence the action, efficacy, and stability of this compound. For instance, the presence of other drugs can affect its pharmacokinetics and pharmacodynamics . Additionally, patient-specific factors such as performance status, number of metastatic organs, and response to crizotinib can affect the drug’s progression-free survival . Furthermore, this compound can cause hepatotoxicity, which is associated with oxidative stress and apoptosis .
Biochemical Analysis
Biochemical Properties
Crizotinib-d5 interacts with various enzymes and proteins. It has been shown to inhibit the anaplastic lymphoma kinase (ALK), c-Met, and ROS1 . The nature of these interactions involves binding to the ATP-binding site of these enzymes, thereby inhibiting their activity .
Cellular Effects
This compound has significant effects on various types of cells and cellular processes. It influences cell function by inhibiting the signaling pathways associated with ALK, c-Met, and ROS1 . This inhibition can impact gene expression and cellular metabolism, leading to the death of cancer cells .
Molecular Mechanism
The molecular mechanism of action of this compound involves binding to the ATP-binding site of ALK, c-Met, and ROS1 . This binding inhibits the activity of these enzymes, leading to a decrease in the signaling pathways that they control. This inhibition can lead to changes in gene expression and cellular metabolism .
Temporal Effects in Laboratory Settings
The effects of this compound change over time in laboratory settings. Studies have shown that this compound has a linear two-compartment model and absorption lag time . Over time, the drug’s stability, degradation, and long-term effects on cellular function can be observed .
Dosage Effects in Animal Models
The effects of this compound vary with different dosages in animal models. Higher doses of this compound can lead to toxic or adverse effects
Metabolic Pathways
This compound is involved in various metabolic pathways. It interacts with enzymes such as ALK, c-Met, and ROS1
Properties
IUPAC Name |
3-[(1R)-1-(2,6-dichloro-3-fluorophenyl)ethoxy]-5-[1-(3,3,4,5,5-pentadeuteriopiperidin-4-yl)pyrazol-4-yl]pyridin-2-amine | |
---|---|---|
Details | Computed by Lexichem TK 2.7.0 (PubChem release 2021.08.13) | |
Source | PubChem | |
URL | https://pubchem.ncbi.nlm.nih.gov | |
Description | Data deposited in or computed by PubChem | |
InChI |
InChI=1S/C21H22Cl2FN5O/c1-12(19-16(22)2-3-17(24)20(19)23)30-18-8-13(9-27-21(18)25)14-10-28-29(11-14)15-4-6-26-7-5-15/h2-3,8-12,15,26H,4-7H2,1H3,(H2,25,27)/t12-/m1/s1/i4D2,5D2,15D | |
Details | Computed by InChI 1.0.6 (PubChem release 2021.08.13) | |
Source | PubChem | |
URL | https://pubchem.ncbi.nlm.nih.gov | |
Description | Data deposited in or computed by PubChem | |
InChI Key |
KTEIFNKAUNYNJU-LJIFSARKSA-N | |
Details | Computed by InChI 1.0.6 (PubChem release 2021.08.13) | |
Source | PubChem | |
URL | https://pubchem.ncbi.nlm.nih.gov | |
Description | Data deposited in or computed by PubChem | |
Canonical SMILES |
CC(C1=C(C=CC(=C1Cl)F)Cl)OC2=C(N=CC(=C2)C3=CN(N=C3)C4CCNCC4)N | |
Details | Computed by OEChem 2.3.0 (PubChem release 2021.08.13) | |
Source | PubChem | |
URL | https://pubchem.ncbi.nlm.nih.gov | |
Description | Data deposited in or computed by PubChem | |
Isomeric SMILES |
[2H]C1(CNCC(C1([2H])N2C=C(C=N2)C3=CC(=C(N=C3)N)O[C@H](C)C4=C(C=CC(=C4Cl)F)Cl)([2H])[2H])[2H] | |
Details | Computed by OEChem 2.3.0 (PubChem release 2021.08.13) | |
Source | PubChem | |
URL | https://pubchem.ncbi.nlm.nih.gov | |
Description | Data deposited in or computed by PubChem | |
Molecular Formula |
C21H22Cl2FN5O | |
Details | Computed by PubChem 2.1 (PubChem release 2021.08.13) | |
Source | PubChem | |
URL | https://pubchem.ncbi.nlm.nih.gov | |
Description | Data deposited in or computed by PubChem | |
Molecular Weight |
455.4 g/mol | |
Details | Computed by PubChem 2.1 (PubChem release 2021.08.13) | |
Source | PubChem | |
URL | https://pubchem.ncbi.nlm.nih.gov | |
Description | Data deposited in or computed by PubChem | |
Retrosynthesis Analysis
AI-Powered Synthesis Planning: Our tool employs the Template_relevance Pistachio, Template_relevance Bkms_metabolic, Template_relevance Pistachio_ringbreaker, Template_relevance Reaxys, Template_relevance Reaxys_biocatalysis model, leveraging a vast database of chemical reactions to predict feasible synthetic routes.
One-Step Synthesis Focus: Specifically designed for one-step synthesis, it provides concise and direct routes for your target compounds, streamlining the synthesis process.
Accurate Predictions: Utilizing the extensive PISTACHIO, BKMS_METABOLIC, PISTACHIO_RINGBREAKER, REAXYS, REAXYS_BIOCATALYSIS database, our tool offers high-accuracy predictions, reflecting the latest in chemical research and data.
Strategy Settings
Precursor scoring | Relevance Heuristic |
---|---|
Min. plausibility | 0.01 |
Model | Template_relevance |
Template Set | Pistachio/Bkms_metabolic/Pistachio_ringbreaker/Reaxys/Reaxys_biocatalysis |
Top-N result to add to graph | 6 |
Feasible Synthetic Routes
Disclaimer and Information on In-Vitro Research Products
Please be aware that all articles and product information presented on BenchChem are intended solely for informational purposes. The products available for purchase on BenchChem are specifically designed for in-vitro studies, which are conducted outside of living organisms. In-vitro studies, derived from the Latin term "in glass," involve experiments performed in controlled laboratory settings using cells or tissues. It is important to note that these products are not categorized as medicines or drugs, and they have not received approval from the FDA for the prevention, treatment, or cure of any medical condition, ailment, or disease. We must emphasize that any form of bodily introduction of these products into humans or animals is strictly prohibited by law. It is essential to adhere to these guidelines to ensure compliance with legal and ethical standards in research and experimentation.