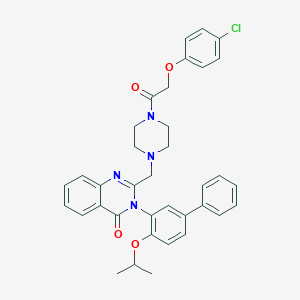
Erastin2
Overview
Description
Erastin2 is a compound that falls within the realm of ferroptosis inducers. It selectively inhibits the system xc (-) cystine/glutamate transporter, making it a potent player in cell death pathways . Unlike its predecessor, Erastin, this compound boasts improved inhibitory potency and ferroptosis induction efficacy .
Mechanism of Action
Target of Action
Erastin2 is a potent, selective inhibitor of the system xc- cystine/glutamate transporter . This transporter plays a crucial role in maintaining the balance of cystine and glutamate in cells . By inhibiting this transporter, this compound disrupts this balance, leading to a series of biochemical reactions that result in cell death .
Mode of Action
This compound interacts with its primary target, the system xc- cystine/glutamate transporter, and inhibits its function . This inhibition leads to a decrease in the uptake of cystine, an essential amino acid for the synthesis of glutathione (GSH), a major cellular antioxidant . The resulting decrease in GSH levels leads to an increase in reactive oxygen species (ROS) within the cell .
Biochemical Pathways
The primary biochemical pathway affected by this compound is the glutathione (GSH) pathway . GSH is a major cellular antioxidant that protects cells from oxidative damage . By inhibiting the uptake of cystine, this compound reduces the synthesis of GSH, leading to an increase in ROS . This increase in ROS triggers a form of cell death known as ferroptosis . The erastin-induced ferroptosis in cells is probably mediated via activation of JNK/P38/MAPK pathways and upregulation of NOX4 expression .
Pharmacokinetics
It is known that this compound has low solubility and poor metabolic stability in a mouse liver microsome assay . These properties could potentially affect the bioavailability of this compound, limiting its effectiveness .
Result of Action
The primary result of this compound’s action is the induction of ferroptosis, a form of cell death characterized by iron-dependent lipid peroxidation . This process is distinct from other forms of cell death such as apoptosis and autophagy . In cancer cells, the induction of ferroptosis by this compound can lead to a decrease in cell viability and an increase in cell death .
Action Environment
The action of this compound can be influenced by various environmental factors. For example, the presence of high concentrations of antioxidants in cells can be a significant obstacle in the effectiveness of this compound . Additionally, the physiological and pathological changes in healthy tissues can also influence the action of this compound .
Biochemical Analysis
Biochemical Properties
Erastin2 interacts with the system xc-, inhibiting the exchange of extracellular cystine for intracellular glutamate . This inhibition leads to a decrease in intracellular cystine, which is crucial for the synthesis of glutathione (GSH), a major cellular antioxidant . The reduction in GSH levels disrupts the cellular redox balance, leading to the accumulation of reactive oxygen species (ROS) and lipid peroxidation, which are key features of ferroptosis .
Cellular Effects
This compound has been shown to induce ferroptosis in various types of cells, including cancer cells . It decreases the concentration of glutathione and the expression of glutathione peroxidase 4 (GPX4), a key enzyme in preventing lipid peroxidation . Moreover, this compound enhances the sensitivity of cancer cells to X-ray irradiation, indicating its potential as a radiosensitizer .
Molecular Mechanism
The molecular mechanism of this compound involves the inhibition of the system xc-, leading to glutathione depletion and an increase in lipid peroxidation . This process is facilitated by the binding of this compound to voltage-dependent anion channels (VDAC), reversing tubulin’s inhibition on VDAC2 and VDAC3 . The resulting oxidative stress triggers ferroptosis, a form of cell death distinct from apoptosis and autophagy .
Temporal Effects in Laboratory Settings
Over time, the effects of this compound can lead to significant changes in cellular function. For example, it has been shown to enhance X-ray irradiation-induced cell death in human tumor cell lines . Additionally, this compound has been observed to cause mild cerebral infarction of the brain and duodenal epithelium hyperplasia in mice .
Dosage Effects in Animal Models
In animal models, the effects of this compound vary with different dosages. For instance, in a tumor-transplanted mouse model, this compound demonstrated a radiosensitizing effect and decreased intratumoral glutathione concentration .
Metabolic Pathways
This compound is involved in the cystine/glutamate antiporter system xc-, a key component of the cellular antioxidant defense system . By inhibiting this system, this compound disrupts the synthesis of glutathione, leading to an increase in oxidative stress and the induction of ferroptosis .
Transport and Distribution
While specific transporters or binding proteins for this compound have not been identified, its effects on cellular function suggest that it is able to penetrate cellular membranes and exert its effects intracellularly .
Subcellular Localization
Given its mechanism of action, it is likely that this compound localizes to areas where the system xc- is present, such as the plasma membrane
Preparation Methods
Industrial Production:: Custom synthesis services may be employed to obtain this compound promptly .
Chemical Reactions Analysis
Erastin2’s reactivity centers around its role as a ferroptosis inducer. While specific reactions are not explicitly outlined, it likely undergoes processes related to iron metabolism and oxidative stress. Common reagents and conditions remain elusive, but further investigation is warranted.
Scientific Research Applications
Erastin2 finds applications across various scientific domains:
Chemistry: Researchers explore its interactions with cellular components, including the cystine/glutamate transporter.
Biology: this compound aids in understanding cell death pathways, particularly ferroptosis.
Medicine: Its potential therapeutic applications include neurodegenerative diseases, where ferroptosis plays a role.
Industry: Although not directly used in industry, insights from this compound research may inform drug development and iron-related processes.
Comparison with Similar Compounds
Erastin2 stands out due to its enhanced potency compared to Erastin. Similar compounds include:
Erastin: The predecessor, less potent than this compound.
Ferrostatins: These antioxidants counteract ferroptosis by trapping lipid peroxides.
Properties
IUPAC Name |
2-[[4-[2-(4-chlorophenoxy)acetyl]piperazin-1-yl]methyl]-3-(5-phenyl-2-propan-2-yloxyphenyl)quinazolin-4-one | |
---|---|---|
Details | Computed by Lexichem TK 2.7.0 (PubChem release 2021.05.07) | |
Source | PubChem | |
URL | https://pubchem.ncbi.nlm.nih.gov | |
Description | Data deposited in or computed by PubChem | |
InChI |
InChI=1S/C36H35ClN4O4/c1-25(2)45-33-17-12-27(26-8-4-3-5-9-26)22-32(33)41-34(38-31-11-7-6-10-30(31)36(41)43)23-39-18-20-40(21-19-39)35(42)24-44-29-15-13-28(37)14-16-29/h3-17,22,25H,18-21,23-24H2,1-2H3 | |
Details | Computed by InChI 1.0.6 (PubChem release 2021.05.07) | |
Source | PubChem | |
URL | https://pubchem.ncbi.nlm.nih.gov | |
Description | Data deposited in or computed by PubChem | |
InChI Key |
WLPHOTYCGOGILE-UHFFFAOYSA-N | |
Details | Computed by InChI 1.0.6 (PubChem release 2021.05.07) | |
Source | PubChem | |
URL | https://pubchem.ncbi.nlm.nih.gov | |
Description | Data deposited in or computed by PubChem | |
Canonical SMILES |
CC(C)OC1=C(C=C(C=C1)C2=CC=CC=C2)N3C(=NC4=CC=CC=C4C3=O)CN5CCN(CC5)C(=O)COC6=CC=C(C=C6)Cl | |
Details | Computed by OEChem 2.3.0 (PubChem release 2021.05.07) | |
Source | PubChem | |
URL | https://pubchem.ncbi.nlm.nih.gov | |
Description | Data deposited in or computed by PubChem | |
Molecular Formula |
C36H35ClN4O4 | |
Details | Computed by PubChem 2.1 (PubChem release 2021.05.07) | |
Source | PubChem | |
URL | https://pubchem.ncbi.nlm.nih.gov | |
Description | Data deposited in or computed by PubChem | |
Molecular Weight |
623.1 g/mol | |
Details | Computed by PubChem 2.1 (PubChem release 2021.05.07) | |
Source | PubChem | |
URL | https://pubchem.ncbi.nlm.nih.gov | |
Description | Data deposited in or computed by PubChem | |
Retrosynthesis Analysis
AI-Powered Synthesis Planning: Our tool employs the Template_relevance Pistachio, Template_relevance Bkms_metabolic, Template_relevance Pistachio_ringbreaker, Template_relevance Reaxys, Template_relevance Reaxys_biocatalysis model, leveraging a vast database of chemical reactions to predict feasible synthetic routes.
One-Step Synthesis Focus: Specifically designed for one-step synthesis, it provides concise and direct routes for your target compounds, streamlining the synthesis process.
Accurate Predictions: Utilizing the extensive PISTACHIO, BKMS_METABOLIC, PISTACHIO_RINGBREAKER, REAXYS, REAXYS_BIOCATALYSIS database, our tool offers high-accuracy predictions, reflecting the latest in chemical research and data.
Strategy Settings
Precursor scoring | Relevance Heuristic |
---|---|
Min. plausibility | 0.01 |
Model | Template_relevance |
Template Set | Pistachio/Bkms_metabolic/Pistachio_ringbreaker/Reaxys/Reaxys_biocatalysis |
Top-N result to add to graph | 6 |
Feasible Synthetic Routes
Disclaimer and Information on In-Vitro Research Products
Please be aware that all articles and product information presented on BenchChem are intended solely for informational purposes. The products available for purchase on BenchChem are specifically designed for in-vitro studies, which are conducted outside of living organisms. In-vitro studies, derived from the Latin term "in glass," involve experiments performed in controlled laboratory settings using cells or tissues. It is important to note that these products are not categorized as medicines or drugs, and they have not received approval from the FDA for the prevention, treatment, or cure of any medical condition, ailment, or disease. We must emphasize that any form of bodily introduction of these products into humans or animals is strictly prohibited by law. It is essential to adhere to these guidelines to ensure compliance with legal and ethical standards in research and experimentation.