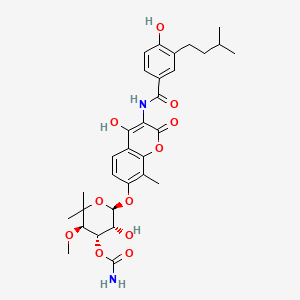
Dihydronovobiocin
Overview
Description
Dihydronovobiocin is a hydrogenated derivative of the coumarin antibiotic novobiocin, synthesized via catalytic hydrogenation . Structurally, it retains the 4-hydroxy coumarin core but features a reduced side chain, enhancing its binding affinity to specific protein targets. Originally developed as an antibacterial agent, this compound inhibits DNA gyrase subunit B (IC50 = 64.5 nM) and exhibits activity against Gram-positive bacteria such as Staphylococcus aureus (MIC = 0.6 µg/ml) and Streptococcus pneumoniae (MIC = 0.6 µg/ml) . However, its clinical use as an antibiotic has declined due to the availability of more effective alternatives .
Recent research highlights its role in modulating autophagy-related protein-protein interactions (PPIs). This compound binds selectively to LC3A and LC3B adapter proteins, key components of the autophagy machinery, by occupying the hydrophobic pocket 2 (HP2) of their LIR-docking sites. This interaction disrupts the LC3-p62 interface, making it a promising candidate for autophagy-mediated therapeutic strategies .
Preparation Methods
Synthetic Routes and Reaction Conditions: The synthesis of dihydronovobiocin involves the hydrogenation of novobiocin. The process typically includes dissolving novobiocin in a suitable solvent, such as ethanol or methanol, and then subjecting it to hydrogenation using a palladium catalyst under controlled conditions . The reaction is carried out at room temperature and atmospheric pressure until the desired level of hydrogenation is achieved.
Industrial Production Methods: Industrial production of this compound follows a similar synthetic route but on a larger scale. The process involves the use of large reactors and continuous hydrogenation systems to ensure consistent product quality and yield. The reaction conditions, such as temperature, pressure, and catalyst concentration, are optimized to maximize the efficiency of the process .
Chemical Reactions Analysis
Types of Reactions: Dihydronovobiocin undergoes several types of chemical reactions, including:
Oxidation: this compound can be oxidized to form various oxidation products. Common oxidizing agents include hydrogen peroxide and potassium permanganate.
Reduction: The compound can be further reduced to form more hydrogenated derivatives.
Substitution: this compound can undergo substitution reactions, particularly at the hydroxyl and amide groups.
Common Reagents and Conditions:
Oxidation: Hydrogen peroxide in an acidic medium or potassium permanganate in a neutral or slightly alkaline medium.
Reduction: Hydrogen gas in the presence of a palladium catalyst.
Substitution: Various nucleophiles, such as amines or alcohols, under acidic or basic conditions.
Major Products Formed:
Oxidation: Formation of hydroxylated or ketone derivatives.
Reduction: Formation of more hydrogenated derivatives.
Substitution: Formation of substituted amides or ethers.
Scientific Research Applications
Antimicrobial Activity
Overview:
Dihydronovobiocin exhibits potent antimicrobial properties, particularly against Gram-positive bacteria. Its mechanism of action primarily involves inhibiting bacterial DNA gyrase, an essential enzyme for DNA replication.
Case Studies:
- In Vitro Studies: Research has demonstrated that DHNB is effective against strains of Staphylococcus aureus and Streptococcus pneumoniae, showcasing its potential as an alternative treatment for infections caused by antibiotic-resistant bacteria .
- Synergistic Effects: Studies have indicated that DHNB can enhance the efficacy of other antibiotics when used in combination therapies, potentially reducing the required dosages and minimizing side effects .
Anticancer Properties
Overview:
Recent investigations have highlighted the anticancer potential of this compound, particularly in targeting cancer cells through apoptosis induction and cell cycle arrest.
Case Studies:
- Cell Line Studies: In vitro studies on various cancer cell lines, including breast and prostate cancer cells, have shown that DHNB can inhibit cell proliferation and induce apoptosis at specific concentrations .
- Animal Models: Preclinical trials using animal models have reported significant tumor suppression when DHNB is administered, indicating its potential for development as a chemotherapeutic agent .
Research in Drug Development
Overview:
this compound is being explored as a lead compound in drug development due to its unique structural attributes and biological activities.
Applications:
- Lead Compound for Derivatives: Researchers are investigating modifications of DHNB to enhance its pharmacological properties and reduce toxicity. These derivatives may have improved efficacy against resistant bacterial strains or cancer cells .
- Formulation Development: The formulation of DHNB into nanoparticles is being studied to improve bioavailability and targeted delivery systems for both antimicrobial and anticancer therapies.
Potential in Veterinary Medicine
Overview:
The veterinary applications of this compound are also being explored, particularly for treating infections in livestock and pets.
Case Studies:
- Livestock Health: Preliminary studies suggest that DHNB could be effective against common bacterial infections in cattle and poultry, potentially reducing reliance on conventional antibiotics.
- Pet Treatments: Research into the use of DHNB for treating infections in companion animals is ongoing, with promising results in preliminary trials.
Mechanistic Insights
Understanding the molecular mechanisms underlying the actions of this compound is crucial for optimizing its applications:
Mechanism | Description |
---|---|
DNA Gyrase Inhibition | DHNB binds to DNA gyrase, preventing DNA replication in bacteria. |
Induction of Apoptosis | Triggers programmed cell death pathways in malignant cells. |
Synergistic Interactions | Enhances the effectiveness of existing antibiotics through combination therapy. |
Mechanism of Action
Dihydronovobiocin exerts its antibacterial effects by inhibiting the DNA gyrase subunit B, an essential enzyme in bacterial DNA replication . By binding to this subunit, this compound blocks the adenosine triphosphatase (ATPase) activity, which is crucial for the energy transduction required for DNA supercoiling . This inhibition prevents the bacteria from replicating their DNA, ultimately leading to cell death .
Comparison with Similar Compounds
Comparison with Similar Compounds
Structural and Functional Analogues
Novobiocin
- Structure: Shares the 4-hydroxy coumarin scaffold but lacks the hydrogenated side chain present in dihydronovobiocin.
- Mechanism: Binds LC3B via HP2 but with lower potency (IC50 = 72.3 ± 7.4 mmol/L for LC3B vs. This compound’s IC50 = 16.0 ± 1.0 mmol/L for LC3A) .
- Applications : Primarily used historically as an antibiotic; less effective in autophagy modulation compared to its reduced derivative .
Chlorobiocin
- Structure : Contains a chlorine substituent on the coumarin ring, altering electronic properties.
- Mechanism: Inhibits DNA gyrase but with a distinct binding mode. No reported activity on LC3 proteins.
Tetracycline
- Structure : A tetracyclic naphthacene carboxamide, structurally unrelated to coumarins.
- Mechanism : Broad-spectrum antibiotic inhibiting bacterial protein synthesis.
Binding and Potency Profiles
*MIC values vary by bacterial strain.
Mechanistic Differentiation
- Binding Specificity: this compound occupies HP2 of LC3A/B via hydrophobic interactions and hydrogen bonding with residues K49 and L53, mimicking the leucine residue of p62’s LIR motif. Novobiocin, while binding HP2, shows weaker occupancy and lacks crystallographic evidence of LC3A interaction .
- Autophagy Modulation: this compound is the first nonpeptide inhibitor of LC3A/B PPIs, laying the groundwork for autophagy-mediated degraders (AUTACs).
- Antibacterial Spectrum: this compound and novobiocin are ineffective against enterobacteria, whereas tetracycline and chlorobiocin exhibit broader activity .
Biological Activity
Dihydronovobiocin, a derivative of novobiocin, has garnered attention for its biological activities, particularly as an antibacterial agent and its potential role in targeting autophagy-related proteins. This article provides an in-depth analysis of the compound's biological activity, supported by data tables and relevant research findings.
This compound is structurally similar to novobiocin, which is known for its ability to inhibit bacterial DNA gyrase and topoisomerase IV. These enzymes are crucial for bacterial DNA replication and transcription. The inhibition of these targets leads to the cessation of bacterial growth and replication.
Antibacterial Activity
The antibacterial efficacy of this compound has been evaluated against various strains of bacteria. The minimal inhibitory concentrations (MICs) provide insight into its potency compared to other antibiotics.
Table 1: Antibacterial Activity of this compound
Bacterial Strain | MIC (µg/mL) |
---|---|
Staphylococcus aureus | 0.1 |
Streptococcus pneumoniae | 0.4 |
Escherichia coli | 6.2 |
Klebsiella pneumoniae | 12.5 |
These results indicate that this compound exhibits strong antibacterial activity, particularly against Staphylococcus aureus, which is significant given the increasing resistance to conventional antibiotics.
Binding Affinity to LC3 Proteins
Recent studies have highlighted the potential of this compound as a ligand for LC3A and LC3B proteins, which are involved in autophagy processes. The binding affinity of this compound was assessed through various assays.
Table 2: Binding Affinity of this compound to LC3 Proteins
Protein | Binding Affinity (K, µM) |
---|---|
LC3A | 17.4 |
LC3B | 48.4 |
The results demonstrate that this compound binds with moderate affinity to these proteins, suggesting a potential role in modulating autophagy pathways, which could be beneficial in cancer therapy and other diseases where autophagy plays a critical role .
Case Studies and Research Findings
- In Vivo Efficacy : In animal models, this compound has shown promising results against infections caused by resistant strains of bacteria. A study indicated that treatment with this compound led to a significant reduction in bacterial load compared to untreated controls .
- Combination Therapies : Research has explored the use of this compound in combination with other antibiotics to enhance therapeutic efficacy. For instance, when combined with beta-lactams, it demonstrated a synergistic effect against resistant Staphylococcus aureus strains .
- Pharmacokinetics : Studies on the pharmacokinetic profile of this compound indicate favorable absorption characteristics, making it a candidate for oral administration. Its bioavailability is significantly improved compared to novobiocin, allowing for effective systemic concentrations .
Q & A
Basic Research Questions
Q. What experimental methods are used to identify dihydronovobiocin’s mechanism of action in targeting LC3 proteins?
- Methodological Answer : Utilize X-ray crystallography to resolve the binding interface between this compound and LC3A, as demonstrated in structural studies . Pair this with competitive binding assays (e.g., AlphaScreen technology) to quantify interactions with LC3 isoforms. Validate findings using site-directed mutagenesis of key residues (e.g., HP2’s L-site) to confirm specificity .
Q. How should researchers design experiments to test this compound’s bioactivity in autophagy-related pathways?
- Methodological Answer : Employ high-throughput screening with counter-assays to eliminate false positives (e.g., interference with SQSTM1/p62 peptide binding). Use cell-based autophagy flux assays (e.g., LC3-II turnover via immunoblotting) and correlate results with in vitro binding data. Adhere to NIH preclinical reporting guidelines to ensure reproducibility .
Q. What are the key assays for validating this compound’s selectivity across LC3 protein isoforms?
- Methodological Answer : Perform isothermal titration calorimetry (ITC) or surface plasmon resonance (SPR) to measure binding affinities (Kd) for LC3A, LC3B, and GABARAP isoforms. Cross-validate with cellular colocalization studies using fluorescence-tagged LC3 constructs .
Advanced Research Questions
Q. How can researchers resolve contradictions between in vitro binding data and cellular efficacy of this compound analogs?
- Methodological Answer : Conduct pharmacokinetic/pharmacodynamic (PK/PD) modeling to assess membrane permeability and intracellular concentrations. Use proteolysis-targeting chimeras (PROTACs) to enhance target engagement or apply chemical biology tools (e.g., photoaffinity labeling) to track compound localization in live cells .
Q. How can researchers address variability in this compound’s activity due to cellular context (e.g., cancer vs. normal cells)?
- Methodological Answer : Perform multi-omics integration (proteomics, metabolomics) to identify context-dependent biomarkers. Use patient-derived organoids or co-culture systems to mimic tissue microenvironments. Apply machine learning classifiers to predict responsive subpopulations .
Q. Ethical & Methodological Compliance
Q. What ethical considerations apply to preclinical studies involving this compound?
- Methodological Answer : Follow ARRIVE 2.0 guidelines for animal studies, including randomization, blinding, and sample-size justification. Disclose conflicts of interest and obtain institutional approval for human-derived cell lines .
Q. How should researchers handle discrepancies between computational predictions and experimental results for this compound analogs?
- Methodological Answer : Re-evaluate force field parameters in docking simulations or use ensemble docking to account for protein flexibility. Validate with crystallographic fragment screening or NMR-based ligand-observed experiments .
Properties
IUPAC Name |
[(3R,4S,5R,6R)-5-hydroxy-6-[4-hydroxy-3-[[4-hydroxy-3-(3-methylbutyl)benzoyl]amino]-8-methyl-2-oxochromen-7-yl]oxy-3-methoxy-2,2-dimethyloxan-4-yl] carbamate | |
---|---|---|
Details | Computed by Lexichem TK 2.7.0 (PubChem release 2021.05.07) | |
Source | PubChem | |
URL | https://pubchem.ncbi.nlm.nih.gov | |
Description | Data deposited in or computed by PubChem | |
InChI |
InChI=1S/C31H38N2O11/c1-14(2)7-8-16-13-17(9-11-19(16)34)27(37)33-21-22(35)18-10-12-20(15(3)24(18)42-28(21)38)41-29-23(36)25(43-30(32)39)26(40-6)31(4,5)44-29/h9-14,23,25-26,29,34-36H,7-8H2,1-6H3,(H2,32,39)(H,33,37)/t23-,25+,26-,29-/m1/s1 | |
Details | Computed by InChI 1.0.6 (PubChem release 2021.05.07) | |
Source | PubChem | |
URL | https://pubchem.ncbi.nlm.nih.gov | |
Description | Data deposited in or computed by PubChem | |
InChI Key |
JJYCENBZIMIWTM-KGSXXDOSSA-N | |
Details | Computed by InChI 1.0.6 (PubChem release 2021.05.07) | |
Source | PubChem | |
URL | https://pubchem.ncbi.nlm.nih.gov | |
Description | Data deposited in or computed by PubChem | |
Canonical SMILES |
CC1=C(C=CC2=C1OC(=O)C(=C2O)NC(=O)C3=CC(=C(C=C3)O)CCC(C)C)OC4C(C(C(C(O4)(C)C)OC)OC(=O)N)O | |
Details | Computed by OEChem 2.3.0 (PubChem release 2021.05.07) | |
Source | PubChem | |
URL | https://pubchem.ncbi.nlm.nih.gov | |
Description | Data deposited in or computed by PubChem | |
Isomeric SMILES |
CC1=C(C=CC2=C1OC(=O)C(=C2O)NC(=O)C3=CC(=C(C=C3)O)CCC(C)C)O[C@H]4[C@@H]([C@@H]([C@H](C(O4)(C)C)OC)OC(=O)N)O | |
Details | Computed by OEChem 2.3.0 (PubChem release 2021.05.07) | |
Source | PubChem | |
URL | https://pubchem.ncbi.nlm.nih.gov | |
Description | Data deposited in or computed by PubChem | |
Molecular Formula |
C31H38N2O11 | |
Details | Computed by PubChem 2.1 (PubChem release 2021.05.07) | |
Source | PubChem | |
URL | https://pubchem.ncbi.nlm.nih.gov | |
Description | Data deposited in or computed by PubChem | |
DSSTOX Substance ID |
DTXSID601316470 | |
Record name | Dihydronovobiocin | |
Source | EPA DSSTox | |
URL | https://comptox.epa.gov/dashboard/DTXSID601316470 | |
Description | DSSTox provides a high quality public chemistry resource for supporting improved predictive toxicology. | |
Molecular Weight |
614.6 g/mol | |
Details | Computed by PubChem 2.1 (PubChem release 2021.05.07) | |
Source | PubChem | |
URL | https://pubchem.ncbi.nlm.nih.gov | |
Description | Data deposited in or computed by PubChem | |
CAS No. |
29826-16-2 | |
Record name | Dihydronovobiocin | |
Source | CAS Common Chemistry | |
URL | https://commonchemistry.cas.org/detail?cas_rn=29826-16-2 | |
Description | CAS Common Chemistry is an open community resource for accessing chemical information. Nearly 500,000 chemical substances from CAS REGISTRY cover areas of community interest, including common and frequently regulated chemicals, and those relevant to high school and undergraduate chemistry classes. This chemical information, curated by our expert scientists, is provided in alignment with our mission as a division of the American Chemical Society. | |
Explanation | The data from CAS Common Chemistry is provided under a CC-BY-NC 4.0 license, unless otherwise stated. | |
Record name | Dihydronovobiocin | |
Source | ChemIDplus | |
URL | https://pubchem.ncbi.nlm.nih.gov/substance/?source=chemidplus&sourceid=0029826162 | |
Description | ChemIDplus is a free, web search system that provides access to the structure and nomenclature authority files used for the identification of chemical substances cited in National Library of Medicine (NLM) databases, including the TOXNET system. | |
Record name | Dihydronovobiocin | |
Source | EPA DSSTox | |
URL | https://comptox.epa.gov/dashboard/DTXSID601316470 | |
Description | DSSTox provides a high quality public chemistry resource for supporting improved predictive toxicology. | |
Retrosynthesis Analysis
AI-Powered Synthesis Planning: Our tool employs the Template_relevance Pistachio, Template_relevance Bkms_metabolic, Template_relevance Pistachio_ringbreaker, Template_relevance Reaxys, Template_relevance Reaxys_biocatalysis model, leveraging a vast database of chemical reactions to predict feasible synthetic routes.
One-Step Synthesis Focus: Specifically designed for one-step synthesis, it provides concise and direct routes for your target compounds, streamlining the synthesis process.
Accurate Predictions: Utilizing the extensive PISTACHIO, BKMS_METABOLIC, PISTACHIO_RINGBREAKER, REAXYS, REAXYS_BIOCATALYSIS database, our tool offers high-accuracy predictions, reflecting the latest in chemical research and data.
Strategy Settings
Precursor scoring | Relevance Heuristic |
---|---|
Min. plausibility | 0.01 |
Model | Template_relevance |
Template Set | Pistachio/Bkms_metabolic/Pistachio_ringbreaker/Reaxys/Reaxys_biocatalysis |
Top-N result to add to graph | 6 |
Feasible Synthetic Routes
Disclaimer and Information on In-Vitro Research Products
Please be aware that all articles and product information presented on BenchChem are intended solely for informational purposes. The products available for purchase on BenchChem are specifically designed for in-vitro studies, which are conducted outside of living organisms. In-vitro studies, derived from the Latin term "in glass," involve experiments performed in controlled laboratory settings using cells or tissues. It is important to note that these products are not categorized as medicines or drugs, and they have not received approval from the FDA for the prevention, treatment, or cure of any medical condition, ailment, or disease. We must emphasize that any form of bodily introduction of these products into humans or animals is strictly prohibited by law. It is essential to adhere to these guidelines to ensure compliance with legal and ethical standards in research and experimentation.