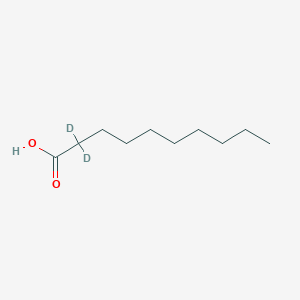
Decanoic-2,2-D2 acid
Overview
Description
Decanoic-2,2-D2 acid, also known as α-Dideuterodecanoic acid or Decanoic acid-2,2-d2, is a product in the category of Stable Isotope Labeled (SIL) Fatty Acids and Esters . It has a molecular formula of C10H18D2O2 and a molecular weight of 174.28 . It is supplied as a neat liquid at room temperature . It is a deuterium-labeled version of Decanoic acid, which is a component of medium-chain triglycerides and has antiseizure effects .
Synthesis Analysis
Decanoic acid, from which Decanoic-2,2-D2 acid is derived, is primarily produced industrially through the oxidation of the corresponding aldehyde, decanal . The aldehyde can be obtained by the hydroformylation of 1-nonene .Molecular Structure Analysis
The molecular formula of Decanoic-2,2-D2 acid is C10H18D2O2 . The average mass of Decanoic acid is 172.265 Da and the monoisotopic mass is 172.146332 Da . The structure of Decanoic acid is a white, crystalline substance at room temperature and pressure .Chemical Reactions Analysis
Decanoic acid, the non-deuterated form of Decanoic-2,2-D2 acid, has been studied for its reactions. For instance, it has been used in the esterification reaction with ethanol in the presence of a solid catalyst Amberlyst 15 .Physical And Chemical Properties Analysis
Decanoic acid is a white, crystalline substance at room temperature and pressure . It has a strong, unpleasant odor that is often compared to that of sweaty socks or goat . It is capable of forming hydrogen bonds, contributing to its relative stability and its characteristic sharp, sour taste . It is soluble in alcohol, ether and slightly soluble in water .Scientific Research Applications
Metabolic Studies
Decanoic-2,2-D2 acid, a deuterated form of decanoic acid, is extensively used in metabolic studies. The deuterium labeling allows researchers to trace the metabolic pathways and understand the biotransformation of fatty acids in the body. This helps in studying disorders related to fatty acid metabolism and developing targeted therapies .
Neuroscience Research
In neuroscience, Decanoic-2,2-D2 acid is used to investigate the role of fatty acids in brain function. It has been shown to influence neuronal signaling and energy metabolism. Studies have explored its potential in treating neurological disorders such as epilepsy and Alzheimer’s disease by modulating brain energy metabolism and neurotransmitter systems .
Lipidomics
Lipidomics, the large-scale study of pathways and networks of cellular lipids, benefits from the use of Decanoic-2,2-D2 acid. The deuterated compound serves as an internal standard in mass spectrometry, allowing for accurate quantification and analysis of lipid species in biological samples. This is crucial for understanding lipid-related diseases and developing lipid-based biomarkers .
Biofuel Research
Decanoic-2,2-D2 acid is also significant in biofuel research. As a medium-chain fatty acid, it can be used in the production of biodiesel. The deuterium labeling helps in studying the conversion processes and optimizing the production of biofuels from renewable resources. This research is vital for developing sustainable and environmentally friendly energy sources .
Pharmacokinetics
In pharmacokinetic studies, Decanoic-2,2-D2 acid is used to investigate the absorption, distribution, metabolism, and excretion (ADME) of fatty acid-based drugs. The deuterium label allows for precise tracking of the compound in the body, providing valuable data on the drug’s behavior and helping in the design of more effective therapeutic agents .
Nutritional Research
Nutritional research utilizes Decanoic-2,2-D2 acid to study the role of medium-chain fatty acids in diet and health. The compound helps in understanding how these fatty acids are metabolized and their impact on energy balance, weight management, and metabolic health. This research supports the development of dietary recommendations and functional foods .
Environmental Science
In environmental science, Decanoic-2,2-D2 acid is used to study the fate and transport of fatty acids in ecosystems. The deuterium label aids in tracing the degradation and transformation of fatty acids in soil and water, providing insights into their environmental impact and helping in the development of bioremediation strategies .
Biochemical Research
Biochemical research employs Decanoic-2,2-D2 acid to study enzyme kinetics and mechanisms. The deuterium label allows for detailed investigation of enzyme-catalyzed reactions involving fatty acids, helping to elucidate the roles of specific enzymes in metabolic pathways and their potential as drug targets .
Mechanism of Action
Target of Action
Decanoic-2,2-D2 acid, also known as deuterium labeled Decanoic acid or Capric acid, is a medium-chain saturated fatty acid . The proteins that decanoic acid targets include furin, octanoyltransferase, 3-oxoacyl- [acyl-carrier-protein] synthase 1, peptostreptococcal albumin-binding protein, and putative uncharacterized protein tcp14 .
Mode of Action
Decanoic acid is a brain-penetrant and non-competitive inhibitor of AMPA receptor . It interacts with its targets, leading to changes in the function of these proteins. For instance, it inhibits the AMPA receptor, which plays a crucial role in synaptic transmission and plasticity in the central nervous system .
Biochemical Pathways
Decanoic acid is involved in the fatty acid biosynthesis metabolic pathway . It is a component of medium-chain triglycerides and can cross the blood-brain barrier . It is also involved in the metabolism of royal jelly fatty acids in humans .
Result of Action
The molecular and cellular effects of decanoic acid’s action are diverse. It has antiseizure effects , and it’s beneficial in maintaining the health of the skin and hair, and also serves an important role in the production of hormones .
Action Environment
The action, efficacy, and stability of decanoic acid can be influenced by environmental factors. Therefore, the environmental context in which decanoic acid is used or studied can significantly impact its action and effectiveness .
Safety and Hazards
Like most carboxylic acids, decanoic acid is corrosive and can cause burns and eye damage . It is advisable to handle it with appropriate safety measures, including wearing protective clothing, gloves, and eye/face protection . It is harmful if swallowed, and can be irritating to the skin and respiratory system .
Future Directions
Decanoic acid has shown potential in the treatment of certain neurological disorders and is being studied for its role in the ketogenic diet, where it is thought to have antiseizure effects . More recently, the use of decanoic acid in biofuel research has become prominent . In addition, the self-assembly of micelles or vesicles derived from prebiotic amphiphilic molecules like decanoic acid is a cornerstone in the chemical evolution pathway .
properties
IUPAC Name |
2,2-dideuteriodecanoic acid | |
---|---|---|
Details | Computed by LexiChem 2.6.6 (PubChem release 2019.06.18) | |
Source | PubChem | |
URL | https://pubchem.ncbi.nlm.nih.gov | |
Description | Data deposited in or computed by PubChem | |
InChI |
InChI=1S/C10H20O2/c1-2-3-4-5-6-7-8-9-10(11)12/h2-9H2,1H3,(H,11,12)/i9D2 | |
Details | Computed by InChI 1.0.5 (PubChem release 2019.06.18) | |
Source | PubChem | |
URL | https://pubchem.ncbi.nlm.nih.gov | |
Description | Data deposited in or computed by PubChem | |
InChI Key |
GHVNFZFCNZKVNT-KNXIQCGSSA-N | |
Details | Computed by InChI 1.0.5 (PubChem release 2019.06.18) | |
Source | PubChem | |
URL | https://pubchem.ncbi.nlm.nih.gov | |
Description | Data deposited in or computed by PubChem | |
Canonical SMILES |
CCCCCCCCCC(=O)O | |
Details | Computed by OEChem 2.1.5 (PubChem release 2019.06.18) | |
Source | PubChem | |
URL | https://pubchem.ncbi.nlm.nih.gov | |
Description | Data deposited in or computed by PubChem | |
Isomeric SMILES |
[2H]C([2H])(CCCCCCCC)C(=O)O | |
Details | Computed by OEChem 2.1.5 (PubChem release 2019.06.18) | |
Source | PubChem | |
URL | https://pubchem.ncbi.nlm.nih.gov | |
Description | Data deposited in or computed by PubChem | |
Molecular Formula |
C10H20O2 | |
Details | Computed by PubChem 2.1 (PubChem release 2019.06.18) | |
Source | PubChem | |
URL | https://pubchem.ncbi.nlm.nih.gov | |
Description | Data deposited in or computed by PubChem | |
Molecular Weight |
174.28 g/mol | |
Details | Computed by PubChem 2.1 (PubChem release 2021.05.07) | |
Source | PubChem | |
URL | https://pubchem.ncbi.nlm.nih.gov | |
Description | Data deposited in or computed by PubChem | |
Retrosynthesis Analysis
AI-Powered Synthesis Planning: Our tool employs the Template_relevance Pistachio, Template_relevance Bkms_metabolic, Template_relevance Pistachio_ringbreaker, Template_relevance Reaxys, Template_relevance Reaxys_biocatalysis model, leveraging a vast database of chemical reactions to predict feasible synthetic routes.
One-Step Synthesis Focus: Specifically designed for one-step synthesis, it provides concise and direct routes for your target compounds, streamlining the synthesis process.
Accurate Predictions: Utilizing the extensive PISTACHIO, BKMS_METABOLIC, PISTACHIO_RINGBREAKER, REAXYS, REAXYS_BIOCATALYSIS database, our tool offers high-accuracy predictions, reflecting the latest in chemical research and data.
Strategy Settings
Precursor scoring | Relevance Heuristic |
---|---|
Min. plausibility | 0.01 |
Model | Template_relevance |
Template Set | Pistachio/Bkms_metabolic/Pistachio_ringbreaker/Reaxys/Reaxys_biocatalysis |
Top-N result to add to graph | 6 |
Feasible Synthetic Routes
Disclaimer and Information on In-Vitro Research Products
Please be aware that all articles and product information presented on BenchChem are intended solely for informational purposes. The products available for purchase on BenchChem are specifically designed for in-vitro studies, which are conducted outside of living organisms. In-vitro studies, derived from the Latin term "in glass," involve experiments performed in controlled laboratory settings using cells or tissues. It is important to note that these products are not categorized as medicines or drugs, and they have not received approval from the FDA for the prevention, treatment, or cure of any medical condition, ailment, or disease. We must emphasize that any form of bodily introduction of these products into humans or animals is strictly prohibited by law. It is essential to adhere to these guidelines to ensure compliance with legal and ethical standards in research and experimentation.