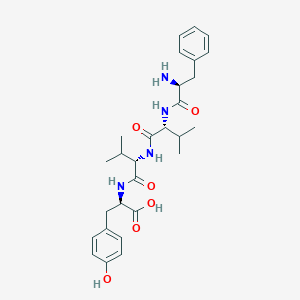
L-Phenylalanyl-D-valyl-L-valyl-D-tyrosine
Overview
Description
Bilaid B: is a tetrapeptide compound with a unique alternating LDLD amino acid configuration. It is structurally similar to opioid peptides but exhibits distinct properties due to its specific chirality. Bilaid B was isolated from an Australian estuarine isolate of the fungus Penicillium species MST-MF667 .
Mechanism of Action
Target of Action:
Bilaid B primarily interacts with the μ-opioid receptor (μ-OR). This receptor is a G protein-coupled receptor (GPCR) found in the central nervous system (CNS) and peripheral tissues. Its role is to modulate pain perception, mood, and other physiological processes .
Mode of Action:
- Unique Structure : Bilaid B’s structure contains an unusual alternating LDLD chirality. This distinct configuration contributes to its interaction with the μ-OR .
Biochemical Pathways:
- Other Effects : While the bioassay profile of Bilaid B remains incompletely investigated, it likely impacts additional pathways .
Pharmacokinetics (ADME Properties):
Action Environment:
Biochemical Analysis
Biochemical Properties
Bilaid B plays a role in biochemical reactions primarily through its weak μ-opioid agonist activity. It interacts with the μ-opioid receptor, albeit with low potency. This interaction is significant as it forms the basis for the development of more potent analogues like bilorphin and bilactorphin . The nature of these interactions involves binding to the receptor, which can influence pain modulation pathways.
Cellular Effects
Bilaid B affects various cell types by modulating cell signaling pathways associated with the μ-opioid receptor. This modulation can influence gene expression and cellular metabolism. For instance, in neuronal cells, Bilaid B’s interaction with the μ-opioid receptor can lead to changes in neurotransmitter release and signal transduction pathways, impacting pain perception and response .
Molecular Mechanism
At the molecular level, Bilaid B exerts its effects by binding to the μ-opioid receptor. This binding can either inhibit or activate downstream signaling pathways, depending on the cellular context. The compound’s weak agonist activity suggests that it may not induce strong conformational changes in the receptor, leading to partial activation of the signaling cascade .
Temporal Effects in Laboratory Settings
In laboratory settings, the effects of Bilaid B can change over time. The compound’s stability and degradation are crucial factors. Bilaid B is stable when stored at -20°C and is soluble in methanol or DMSO . Long-term studies have shown that its weak agonist activity remains consistent, but prolonged exposure can lead to receptor desensitization and altered cellular responses.
Dosage Effects in Animal Models
The effects of Bilaid B vary with different dosages in animal models. At low doses, it exhibits minimal μ-opioid agonist activity, while higher doses can lead to more pronounced effects. Due to its weak potency, even high doses may not produce significant adverse effects. Threshold effects are observed where a certain concentration is required to elicit a measurable response .
Metabolic Pathways
Bilaid B is involved in metabolic pathways related to peptide degradation. Enzymes such as peptidases can cleave the tetrapeptide into smaller fragments, which may then be further metabolized. The interaction with these enzymes can affect the metabolic flux and levels of metabolites within the cell .
Transport and Distribution
Within cells and tissues, Bilaid B is transported and distributed through interactions with specific transporters and binding proteins. These interactions can influence its localization and accumulation in target tissues, such as the nervous system, where the μ-opioid receptors are predominantly expressed .
Subcellular Localization
Bilaid B’s subcellular localization is primarily within the plasma membrane, where it interacts with the μ-opioid receptor. Post-translational modifications and targeting signals may direct it to specific compartments, ensuring its availability for receptor binding and subsequent signaling .
Preparation Methods
Synthetic Routes and Reaction Conditions: Bilaid B can be synthesized through solid-phase peptide synthesis (SPPS), a method commonly used for the production of peptides. The synthesis involves the stepwise addition of amino acids to a growing peptide chain anchored to a solid resin. The specific sequence for Bilaid B is phenylalanine, D-valine, valine, and D-tyrosine .
Industrial Production Methods: Industrial production of Bilaid B involves fermentation processes using the Penicillium species MST-MF667. The fungus is cultured under controlled conditions to produce the tetrapeptide, which is then extracted and purified using high-performance liquid chromatography (HPLC) to achieve a purity of over 95% .
Chemical Reactions Analysis
Types of Reactions: Bilaid B undergoes various chemical reactions, including:
Oxidation: Bilaid B can be oxidized to form hydroxylated derivatives.
Reduction: Reduction reactions can modify the peptide bonds within Bilaid B.
Substitution: Substitution reactions can occur at the amino acid side chains, altering the peptide’s properties
Common Reagents and Conditions:
Oxidation: Hydrogen peroxide or other oxidizing agents under mild conditions.
Reduction: Sodium borohydride or other reducing agents.
Substitution: Various reagents depending on the desired substitution, such as alkyl halides for alkylation
Major Products Formed:
Hydroxylated derivatives: Formed through oxidation.
Reduced peptides: Formed through reduction.
Substituted peptides: Formed through substitution reactions
Scientific Research Applications
Bilaid B has several scientific research applications, including:
Chemistry: Used as a model compound for studying peptide synthesis and modification.
Biology: Investigated for its interactions with opioid receptors and potential as a biased analgesic.
Medicine: Explored for its analgesic properties and potential therapeutic applications.
Industry: Utilized in the development of new peptide-based drugs and as a reference compound in analytical chemistry
Comparison with Similar Compounds
Bilorphin: A more potent analogue of Bilaid B with similar biased agonist properties.
Bilactorphin: Another analogue with enhanced potency and selectivity for the μ-opioid receptor
Uniqueness of Bilaid B: Bilaid B’s unique alternating LDLD chirality sets it apart from other opioid peptides. This specific configuration contributes to its distinct pharmacological profile, making it a valuable compound for research and potential therapeutic applications .
Properties
IUPAC Name |
(2R)-2-[[(2S)-2-[[(2R)-2-[[(2S)-2-amino-3-phenylpropanoyl]amino]-3-methylbutanoyl]amino]-3-methylbutanoyl]amino]-3-(4-hydroxyphenyl)propanoic acid | |
---|---|---|
Details | Computed by Lexichem TK 2.7.0 (PubChem release 2019.06.18) | |
Source | PubChem | |
URL | https://pubchem.ncbi.nlm.nih.gov | |
Description | Data deposited in or computed by PubChem | |
InChI |
InChI=1S/C28H38N4O6/c1-16(2)23(26(35)30-22(28(37)38)15-19-10-12-20(33)13-11-19)32-27(36)24(17(3)4)31-25(34)21(29)14-18-8-6-5-7-9-18/h5-13,16-17,21-24,33H,14-15,29H2,1-4H3,(H,30,35)(H,31,34)(H,32,36)(H,37,38)/t21-,22+,23-,24+/m0/s1 | |
Details | Computed by InChI 1.0.5 (PubChem release 2019.06.18) | |
Source | PubChem | |
URL | https://pubchem.ncbi.nlm.nih.gov | |
Description | Data deposited in or computed by PubChem | |
InChI Key |
LUVNTPPXXIXEDW-UARRHKHWSA-N | |
Details | Computed by InChI 1.0.5 (PubChem release 2019.06.18) | |
Source | PubChem | |
URL | https://pubchem.ncbi.nlm.nih.gov | |
Description | Data deposited in or computed by PubChem | |
Canonical SMILES |
CC(C)C(C(=O)NC(C(C)C)C(=O)NC(CC1=CC=C(C=C1)O)C(=O)O)NC(=O)C(CC2=CC=CC=C2)N | |
Details | Computed by OEChem 2.3.0 (PubChem release 2019.06.18) | |
Source | PubChem | |
URL | https://pubchem.ncbi.nlm.nih.gov | |
Description | Data deposited in or computed by PubChem | |
Isomeric SMILES |
CC(C)[C@H](C(=O)N[C@@H](C(C)C)C(=O)N[C@H](CC1=CC=C(C=C1)O)C(=O)O)NC(=O)[C@H](CC2=CC=CC=C2)N | |
Details | Computed by OEChem 2.3.0 (PubChem release 2019.06.18) | |
Source | PubChem | |
URL | https://pubchem.ncbi.nlm.nih.gov | |
Description | Data deposited in or computed by PubChem | |
Molecular Formula |
C28H38N4O6 | |
Details | Computed by PubChem 2.1 (PubChem release 2019.06.18) | |
Source | PubChem | |
URL | https://pubchem.ncbi.nlm.nih.gov | |
Description | Data deposited in or computed by PubChem | |
Molecular Weight |
526.6 g/mol | |
Details | Computed by PubChem 2.1 (PubChem release 2021.05.07) | |
Source | PubChem | |
URL | https://pubchem.ncbi.nlm.nih.gov | |
Description | Data deposited in or computed by PubChem | |
Retrosynthesis Analysis
AI-Powered Synthesis Planning: Our tool employs the Template_relevance Pistachio, Template_relevance Bkms_metabolic, Template_relevance Pistachio_ringbreaker, Template_relevance Reaxys, Template_relevance Reaxys_biocatalysis model, leveraging a vast database of chemical reactions to predict feasible synthetic routes.
One-Step Synthesis Focus: Specifically designed for one-step synthesis, it provides concise and direct routes for your target compounds, streamlining the synthesis process.
Accurate Predictions: Utilizing the extensive PISTACHIO, BKMS_METABOLIC, PISTACHIO_RINGBREAKER, REAXYS, REAXYS_BIOCATALYSIS database, our tool offers high-accuracy predictions, reflecting the latest in chemical research and data.
Strategy Settings
Precursor scoring | Relevance Heuristic |
---|---|
Min. plausibility | 0.01 |
Model | Template_relevance |
Template Set | Pistachio/Bkms_metabolic/Pistachio_ringbreaker/Reaxys/Reaxys_biocatalysis |
Top-N result to add to graph | 6 |
Feasible Synthetic Routes
Disclaimer and Information on In-Vitro Research Products
Please be aware that all articles and product information presented on BenchChem are intended solely for informational purposes. The products available for purchase on BenchChem are specifically designed for in-vitro studies, which are conducted outside of living organisms. In-vitro studies, derived from the Latin term "in glass," involve experiments performed in controlled laboratory settings using cells or tissues. It is important to note that these products are not categorized as medicines or drugs, and they have not received approval from the FDA for the prevention, treatment, or cure of any medical condition, ailment, or disease. We must emphasize that any form of bodily introduction of these products into humans or animals is strictly prohibited by law. It is essential to adhere to these guidelines to ensure compliance with legal and ethical standards in research and experimentation.