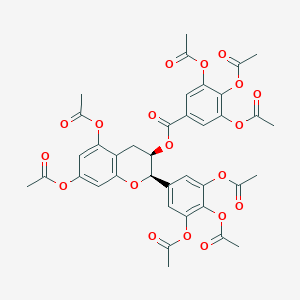
EGCG Octaacetate
Overview
Description
Peracetylated (-)-epigallocatechin-3-gallate, commonly referred to as AcEGCG, is a derivative of epigallocatechin gallate (EGCG), a major polyphenol found in tea. AcEGCG is synthesized to enhance the bioavailability, stability, and liposolubility of EGCG, which is known for its antioxidant, antibacterial, anticancer, and antiviral properties .
Mechanism of Action
Target of Action
EGCG Octaacetate, a prodrug of Epigallocatechin-3-Gallate (EGCG), primarily targets several proteins and enzymes. These include the trans-membrane receptor 67LR, which is a master regulator of many pathways affecting cell proliferation or apoptosis . It also interacts with DNA methyltransferases (DNMTs) and histone deacetylases (HDACs), which modulate epigenetic changes .
Mode of Action
This compound decreases the proinflammatory mediator levels by down-regulating PI3K/Akt/NFκB phosphorylation and p65 acetylation . This interaction results in the inhibition of cell proliferation and promotion of apoptosis, thereby exerting its anti-cancer properties .
Biochemical Pathways
This compound affects multiple crucial cellular signaling pathways, including those mediated by EGFR, JAK-STAT, MAPKs, NF-κB, PI3K-AKT-mTOR, and others . Deregulation of these pathways is involved in the pathophysiology of cancer .
Pharmacokinetics
This compound is utilized to enhance the stability and bioavailability of EGCG in vivo . A study showed that the maximum concentration (Cmax) was 0.067 ± 0.04 μg/mL, the time of maximum concentration (Tmax) was 1.33 h, the area under the curve (AUC) was 0.20 ± 0.05 h × µg/mL, and the elimination rate was 0.20 ± 0.11 hr−1 .
Result of Action
This compound significantly reduces colitis-driven colon cancer in mice . It also significantly decreases the growth of endometrial implants, reduces the lesion size and weight, inhibits functional and structural microvessels in the lesions, and enhances lesion apoptosis .
Action Environment
The action of this compound can be influenced by environmental factors. For instance, due to the hydroxyl structure of EGCG, the further applications of natural EGCG are restricted, which is the poor lipid solubility, unstable in vivo, low absorption, and bioavailability . The acetylation molecular modification can effectively improve the bioavailability and biological activity of EGCG .
Biochemical Analysis
Biochemical Properties
EGCG Octaacetate plays a crucial role in various biochemical reactions. It interacts with several enzymes, proteins, and other biomolecules, modulating their activity and function. For instance, this compound has been shown to down-regulate the phosphorylation of PI3K/Akt/NFκB and the acetylation of p65, leading to a reduction in pro-inflammatory mediator levels . Additionally, this compound exhibits antioxidant properties by scavenging free radicals and inhibiting oxidative stress . These interactions highlight the compound’s potential in modulating biochemical pathways involved in inflammation and oxidative stress.
Cellular Effects
This compound exerts various effects on different types of cells and cellular processes. It influences cell function by modulating cell signaling pathways, gene expression, and cellular metabolism. For example, this compound has been shown to reduce colitis-driven colon cancer in mice by down-regulating pro-inflammatory pathways . It also affects cell signaling pathways such as PI3K/Akt/NFκB, leading to changes in gene expression and cellular responses . Furthermore, this compound’s antioxidant properties help protect cells from oxidative damage, thereby maintaining cellular homeostasis .
Molecular Mechanism
The molecular mechanism of this compound involves its interactions with various biomolecules, leading to changes in their activity and function. This compound binds to and inhibits the activity of enzymes involved in pro-inflammatory pathways, such as PI3K and Akt . This inhibition results in the down-regulation of NFκB phosphorylation and p65 acetylation, reducing the levels of pro-inflammatory mediators . Additionally, this compound’s antioxidant properties are attributed to its ability to scavenge free radicals and inhibit oxidative stress . These molecular interactions highlight the compound’s potential in modulating inflammation and oxidative stress at the molecular level.
Temporal Effects in Laboratory Settings
In laboratory settings, the effects of this compound change over time due to its stability, degradation, and long-term impact on cellular function. This compound is more stable than its parent compound, EGCG, due to the acetylation of its hydroxyl groups . This increased stability allows for prolonged activity and effectiveness in biochemical assays. Over time, this compound may undergo degradation, leading to a reduction in its bioactivity. Long-term studies have shown that this compound can maintain its anti-inflammatory and antioxidant effects in vitro and in vivo, although its efficacy may decrease with prolonged exposure .
Dosage Effects in Animal Models
The effects of this compound vary with different dosages in animal models. At lower doses, this compound exhibits beneficial effects such as reducing inflammation and oxidative stress . At higher doses, the compound may exhibit toxic or adverse effects. For instance, high doses of this compound have been associated with hepatotoxicity and gastrointestinal disturbances in animal models . These findings highlight the importance of determining the optimal dosage for therapeutic applications to maximize benefits while minimizing potential risks.
Metabolic Pathways
This compound is involved in various metabolic pathways, interacting with enzymes and cofactors that modulate its activity and function. The compound undergoes metabolic transformations, including deacetylation, to produce active metabolites such as EGCG diacetates and triacetates . These metabolites retain some of the bioactivity of the parent compound and contribute to its overall therapeutic effects. Additionally, this compound influences metabolic flux and metabolite levels by modulating key enzymes involved in oxidative stress and inflammation .
Transport and Distribution
This compound is transported and distributed within cells and tissues through interactions with transporters and binding proteins. The compound’s acetylated structure enhances its lipid solubility, facilitating its uptake and distribution in cellular membranes . This compound is also known to interact with specific transporters that mediate its cellular uptake and efflux, influencing its localization and accumulation within cells . These interactions play a crucial role in determining the compound’s bioavailability and therapeutic efficacy.
Subcellular Localization
The subcellular localization of this compound is influenced by its chemical structure and interactions with cellular components. This compound is primarily localized in the cytoplasm and cellular membranes, where it exerts its antioxidant and anti-inflammatory effects . The compound’s acetylated structure may also facilitate its targeting to specific organelles, such as the mitochondria, where it can modulate oxidative stress and cellular metabolism . Additionally, post-translational modifications and targeting signals may direct this compound to specific subcellular compartments, influencing its activity and function.
Preparation Methods
The synthesis of AcEGCG involves the acetylation of EGCG. This process can be carried out using both chemical and enzymatic methods.
Chemical Synthesis: This method involves the use of acetic anhydride as the acetylating agent in the presence of a catalyst such as pyridine. The reaction is typically carried out at room temperature for several hours.
Enzymatic Synthesis: This method uses enzymes like lipase to catalyze the acetylation reaction. The reaction is carried out at a controlled temperature (around 45°C) for an extended period (up to 48 hours).
Chemical Reactions Analysis
AcEGCG undergoes various chemical reactions, including:
Oxidation: AcEGCG can undergo oxidation reactions, which may affect its antioxidant properties.
Reduction: Reduction reactions can be carried out using reducing agents like sodium borohydride.
Substitution: AcEGCG can undergo substitution reactions where acetyl groups can be replaced by other functional groups.
Scientific Research Applications
AcEGCG has a wide range of applications in scientific research:
Chemistry: AcEGCG is used as a model compound to study the effects of acetylation on the bioactivity of polyphenols.
Medicine: AcEGCG has shown potential in preventing skin carcinogenesis by inhibiting the PKD1-dependent signaling pathway.
Comparison with Similar Compounds
AcEGCG is compared with other acetylated derivatives of EGCG, such as mono-substituted acetylated EGCG and tri-substituted acetylated EGCG. While all these derivatives aim to improve the bioavailability and stability of EGCG, AcEGCG stands out due to its higher liposolubility and enhanced physiological functions . Similar compounds include:
- Mono-substituted acetylated EGCG
- Tri-substituted acetylated EGCG
- Epigallocatechin gallate (EGCG)
AcEGCG is more effective than EGCG in protecting melanocytes from oxidative damage and treating conditions like vitiligo by inhibiting specific signaling pathways .
Properties
IUPAC Name |
[(2R,3R)-5,7-diacetyloxy-2-(3,4,5-triacetyloxyphenyl)-3,4-dihydro-2H-chromen-3-yl] 3,4,5-triacetyloxybenzoate | |
---|---|---|
Details | Computed by LexiChem 2.6.6 (PubChem release 2019.06.18) | |
Source | PubChem | |
URL | https://pubchem.ncbi.nlm.nih.gov | |
Description | Data deposited in or computed by PubChem | |
InChI |
InChI=1S/C38H34O19/c1-16(39)48-26-13-28(49-17(2)40)27-15-34(57-38(47)25-11-32(52-20(5)43)37(55-23(8)46)33(12-25)53-21(6)44)35(56-29(27)14-26)24-9-30(50-18(3)41)36(54-22(7)45)31(10-24)51-19(4)42/h9-14,34-35H,15H2,1-8H3/t34-,35-/m1/s1 | |
Details | Computed by InChI 1.0.5 (PubChem release 2019.06.18) | |
Source | PubChem | |
URL | https://pubchem.ncbi.nlm.nih.gov | |
Description | Data deposited in or computed by PubChem | |
InChI Key |
SVHJCTSSYQPWEV-VSJLXWSYSA-N | |
Details | Computed by InChI 1.0.5 (PubChem release 2019.06.18) | |
Source | PubChem | |
URL | https://pubchem.ncbi.nlm.nih.gov | |
Description | Data deposited in or computed by PubChem | |
Canonical SMILES |
CC(=O)OC1=CC2=C(CC(C(O2)C3=CC(=C(C(=C3)OC(=O)C)OC(=O)C)OC(=O)C)OC(=O)C4=CC(=C(C(=C4)OC(=O)C)OC(=O)C)OC(=O)C)C(=C1)OC(=O)C | |
Details | Computed by OEChem 2.1.5 (PubChem release 2019.06.18) | |
Source | PubChem | |
URL | https://pubchem.ncbi.nlm.nih.gov | |
Description | Data deposited in or computed by PubChem | |
Isomeric SMILES |
CC(=O)OC1=CC2=C(C[C@H]([C@H](O2)C3=CC(=C(C(=C3)OC(=O)C)OC(=O)C)OC(=O)C)OC(=O)C4=CC(=C(C(=C4)OC(=O)C)OC(=O)C)OC(=O)C)C(=C1)OC(=O)C | |
Details | Computed by OEChem 2.1.5 (PubChem release 2019.06.18) | |
Source | PubChem | |
URL | https://pubchem.ncbi.nlm.nih.gov | |
Description | Data deposited in or computed by PubChem | |
Molecular Formula |
C38H34O19 | |
Details | Computed by PubChem 2.1 (PubChem release 2019.06.18) | |
Source | PubChem | |
URL | https://pubchem.ncbi.nlm.nih.gov | |
Description | Data deposited in or computed by PubChem | |
Molecular Weight |
794.7 g/mol | |
Details | Computed by PubChem 2.1 (PubChem release 2021.05.07) | |
Source | PubChem | |
URL | https://pubchem.ncbi.nlm.nih.gov | |
Description | Data deposited in or computed by PubChem | |
Retrosynthesis Analysis
AI-Powered Synthesis Planning: Our tool employs the Template_relevance Pistachio, Template_relevance Bkms_metabolic, Template_relevance Pistachio_ringbreaker, Template_relevance Reaxys, Template_relevance Reaxys_biocatalysis model, leveraging a vast database of chemical reactions to predict feasible synthetic routes.
One-Step Synthesis Focus: Specifically designed for one-step synthesis, it provides concise and direct routes for your target compounds, streamlining the synthesis process.
Accurate Predictions: Utilizing the extensive PISTACHIO, BKMS_METABOLIC, PISTACHIO_RINGBREAKER, REAXYS, REAXYS_BIOCATALYSIS database, our tool offers high-accuracy predictions, reflecting the latest in chemical research and data.
Strategy Settings
Precursor scoring | Relevance Heuristic |
---|---|
Min. plausibility | 0.01 |
Model | Template_relevance |
Template Set | Pistachio/Bkms_metabolic/Pistachio_ringbreaker/Reaxys/Reaxys_biocatalysis |
Top-N result to add to graph | 6 |
Feasible Synthetic Routes
Q1: What is the mechanism of action of (-)-epigallocatechin-3-gallate (EGCG) octaacetate and how does it differ from its parent compound, EGCG?
A: While both EGCG and its prodrug, EGCG octaacetate, have shown potential anti-cancer activity, their mechanisms differ. This compound acts as a prodrug, meaning it needs to be metabolized in the body to release the active compound, EGCG. [] EGCG itself has been shown to have multiple targets in cancer cells, including inhibiting cell signaling pathways and inducing apoptosis. [] One study suggests that EGCG may exert its anti-cancer effects by targeting the DNA repair enzyme ERCC1/XPF. [] By inhibiting this enzyme, EGCG could potentially enhance the efficacy of DNA-damaging chemotherapy drugs like cisplatin. []
Q2: How does the acetylation of EGCG to form this compound impact its properties and potential applications?
A: The addition of eight acetate groups to EGCG significantly alters its properties. Research suggests that this compound exhibits enhanced lipophilicity compared to EGCG. [] This enhanced lipophilicity may lead to better cell membrane permeability and improved bioavailability, potentially making it a more effective therapeutic agent. [] One study found that this compound demonstrated potent anti-angiogenic activity in a mouse model of endometriosis, suggesting potential therapeutic applications in this area. [, ]
Q3: What is the role of acetate release in the cytotoxic activity of this compound?
A: Interestingly, the acetate groups themselves, when released during the metabolism of this compound, might contribute to the overall cytotoxic effect. Research indicates that acetate at a concentration of 400 μM showed significant growth inhibitory activity against MDAMB-231 breast cancer and SKHep-1 hepatoma cell lines. [] This finding suggests that the release of acetate from this compound could work synergistically with the released EGCG to enhance the overall cytotoxic effect. []
Disclaimer and Information on In-Vitro Research Products
Please be aware that all articles and product information presented on BenchChem are intended solely for informational purposes. The products available for purchase on BenchChem are specifically designed for in-vitro studies, which are conducted outside of living organisms. In-vitro studies, derived from the Latin term "in glass," involve experiments performed in controlled laboratory settings using cells or tissues. It is important to note that these products are not categorized as medicines or drugs, and they have not received approval from the FDA for the prevention, treatment, or cure of any medical condition, ailment, or disease. We must emphasize that any form of bodily introduction of these products into humans or animals is strictly prohibited by law. It is essential to adhere to these guidelines to ensure compliance with legal and ethical standards in research and experimentation.