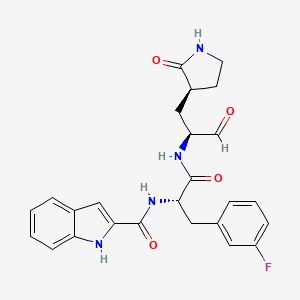
SARS-CoV MPro-IN-1
Overview
Description
MPro Inhibitor 11b is a potent inhibitor of the main protease (MPro) of severe acute respiratory syndrome coronavirus 2 (SARS-CoV-2). This compound has garnered significant attention due to its potential in reducing viral replication and aiding in the treatment of COVID-19 . The main protease is crucial for the viral life cycle, making it an attractive target for antiviral drug development.
Scientific Research Applications
MPro Inhibitor 11b has a wide range of applications in scientific research:
Chemistry: Used as a tool to study the inhibition of proteases and to develop new synthetic methodologies.
Biology: Helps in understanding the role of proteases in viral replication and cellular processes.
Medicine: Potential therapeutic agent for treating COVID-19 by inhibiting the main protease of SARS-CoV-2
Industry: Utilized in the development of antiviral drugs and in the pharmaceutical manufacturing process.
Mechanism of Action
Target of Action
The primary target of the compound SARS-CoV MPro-IN-1 is the main protease (Mpro) of SARS-CoV-2 . Mpro, also known as the 3C-like protease, is a key enzyme involved in the replication process of the virus that is causing the COVID-19 pandemic . It is essential for processing the polyprotein that is translated from viral RNA . The Mpro sequences of SARS-CoV-2 and SARS-CoV-1 are 100% identical in the catalytic domain for protein cleavage , making it a promising target for drug development .
Mode of Action
This compound interacts with its target, the Mpro, through a covalent bond with the thiol of the Cys145 residue . This interaction results in the inhibition of Mpro, thereby preventing the cleavage of the polyprotein necessary for viral replication . The mode of action involves a two-step mechanism, with structures and calculated barriers in good agreement with experiment .
Biochemical Pathways
The inhibition of Mpro affects the viral replication transcription complex (RTC) , which is engaged in RNA synthesis . By preventing the cleavage of the precursor polyproteins into nonstructural proteins, the compound stalls the viral replication cycle . This means that effective inhibition of Mpro activity can stall the viral replication cycle .
Pharmacokinetics
For instance, compound 11a, a potential drug candidate for COVID-19 with strong anti-SARS-CoV-2 infection activity, was designed with good pharmacokinetics characteristics .
Result of Action
The result of the action of this compound is the inhibition of SARS-CoV-2 replication . By binding to the Mpro and preventing the cleavage of the polyprotein, the compound effectively stalls the viral replication cycle . This leads to a reduction in the viral load and potentially alleviates the symptoms of COVID-19 .
Future Directions
The future directions in the research of SARS-CoV MPro-IN-1 involve the development of more efficacious antiviral drug candidates with satisfying drug-likeness properties and safety profiles for combating COVID-19 . The combined inhibition of Mpro and PLpro is more effective in inhibiting SARS-CoV-2 and the delta variant .
Biochemical Analysis
Biochemical Properties
SARS-CoV MPro-IN-1 interacts with the main protease of SARS-CoV-2, inhibiting its function . This interaction is critical as the main protease is responsible for processing the polyproteins that are translated from the viral RNA .
Cellular Effects
The inhibition of the main protease by this compound affects various cellular processes. It disrupts the replication of the virus, thereby influencing cell function . This includes impacts on cell signaling pathways, gene expression, and cellular metabolism .
Molecular Mechanism
This compound exerts its effects at the molecular level through binding interactions with the main protease, leading to its inhibition . This prevents the protease from processing the polyproteins, thereby inhibiting the replication of the virus .
Temporal Effects in Laboratory Settings
The effects of this compound have been observed over time in laboratory settings. Its stability and long-term effects on cellular function have been studied in both in vitro and in vivo studies .
Dosage Effects in Animal Models
The effects of this compound vary with different dosages in animal models . Studies have observed threshold effects, as well as toxic or adverse effects at high doses .
Metabolic Pathways
This compound is involved in the metabolic pathways of the SARS-CoV-2 virus. It interacts with the main protease, a key enzyme in these pathways .
Transport and Distribution
This compound is transported and distributed within cells and tissues. It interacts with the main protease, affecting its localization or accumulation .
Subcellular Localization
The subcellular localization of this compound is associated with its activity or function. It is directed to specific compartments or organelles where it interacts with the main protease .
Preparation Methods
Synthetic Routes and Reaction Conditions: The synthesis of MPro Inhibitor 11b typically involves multiple steps, starting with the preparation of key intermediates. The process often includes:
Formation of the core structure: This involves the use of specific reagents and catalysts to form the backbone of the molecule.
Functional group modifications: Various functional groups are introduced or modified to enhance the compound’s activity and selectivity.
Purification: The final product is purified using techniques such as chromatography to achieve high purity levels.
Industrial Production Methods: In an industrial setting, the production of MPro Inhibitor 11b would be scaled up using optimized synthetic routes. This includes:
Batch processing: Large-scale reactions are conducted in batches to produce significant quantities of the compound.
Continuous flow chemistry: This method allows for the continuous production of the compound, improving efficiency and consistency.
Quality control: Rigorous testing is conducted to ensure the compound meets the required purity and potency standards.
Chemical Reactions Analysis
Types of Reactions: MPro Inhibitor 11b undergoes various chemical reactions, including:
Oxidation: Introduction of oxygen atoms to form oxides.
Reduction: Removal of oxygen atoms or addition of hydrogen atoms.
Substitution: Replacement of one functional group with another.
Common Reagents and Conditions:
Oxidizing agents: Such as potassium permanganate or hydrogen peroxide.
Reducing agents: Such as lithium aluminum hydride or sodium borohydride.
Catalysts: Transition metal catalysts like palladium or platinum are often used.
Major Products: The reactions typically yield modified versions of MPro Inhibitor 11b with enhanced or altered biological activity.
Comparison with Similar Compounds
MPro Inhibitor 11b is compared with other protease inhibitors such as:
Nirmatrelvir: Another potent inhibitor of SARS-CoV-2 main protease.
Boceprevir: Initially developed for hepatitis C virus, also shows activity against SARS-CoV-2 main protease.
GC376: A broad-spectrum antiviral protease inhibitor.
Uniqueness: MPro Inhibitor 11b stands out due to its high potency and selectivity for the SARS-CoV-2 main protease, making it a promising candidate for further development as an antiviral drug .
Properties
IUPAC Name |
N-[(2S)-3-(3-fluorophenyl)-1-oxo-1-[[(2S)-1-oxo-3-[(3S)-2-oxopyrrolidin-3-yl]propan-2-yl]amino]propan-2-yl]-1H-indole-2-carboxamide | |
---|---|---|
Details | Computed by Lexichem TK 2.7.0 (PubChem release 2021.05.07) | |
Source | PubChem | |
URL | https://pubchem.ncbi.nlm.nih.gov | |
Description | Data deposited in or computed by PubChem | |
InChI |
InChI=1S/C25H25FN4O4/c26-18-6-3-4-15(10-18)11-21(24(33)28-19(14-31)12-17-8-9-27-23(17)32)30-25(34)22-13-16-5-1-2-7-20(16)29-22/h1-7,10,13-14,17,19,21,29H,8-9,11-12H2,(H,27,32)(H,28,33)(H,30,34)/t17-,19-,21-/m0/s1 | |
Details | Computed by InChI 1.0.6 (PubChem release 2021.05.07) | |
Source | PubChem | |
URL | https://pubchem.ncbi.nlm.nih.gov | |
Description | Data deposited in or computed by PubChem | |
InChI Key |
HCRBVFBQANEDDM-CUWPLCDZSA-N | |
Details | Computed by InChI 1.0.6 (PubChem release 2021.05.07) | |
Source | PubChem | |
URL | https://pubchem.ncbi.nlm.nih.gov | |
Description | Data deposited in or computed by PubChem | |
Canonical SMILES |
C1CNC(=O)C1CC(C=O)NC(=O)C(CC2=CC(=CC=C2)F)NC(=O)C3=CC4=CC=CC=C4N3 | |
Details | Computed by OEChem 2.3.0 (PubChem release 2021.05.07) | |
Source | PubChem | |
URL | https://pubchem.ncbi.nlm.nih.gov | |
Description | Data deposited in or computed by PubChem | |
Isomeric SMILES |
C1CNC(=O)[C@@H]1C[C@@H](C=O)NC(=O)[C@H](CC2=CC(=CC=C2)F)NC(=O)C3=CC4=CC=CC=C4N3 | |
Details | Computed by OEChem 2.3.0 (PubChem release 2021.05.07) | |
Source | PubChem | |
URL | https://pubchem.ncbi.nlm.nih.gov | |
Description | Data deposited in or computed by PubChem | |
Molecular Formula |
C25H25FN4O4 | |
Details | Computed by PubChem 2.1 (PubChem release 2021.05.07) | |
Source | PubChem | |
URL | https://pubchem.ncbi.nlm.nih.gov | |
Description | Data deposited in or computed by PubChem | |
Molecular Weight |
464.5 g/mol | |
Details | Computed by PubChem 2.1 (PubChem release 2021.05.07) | |
Source | PubChem | |
URL | https://pubchem.ncbi.nlm.nih.gov | |
Description | Data deposited in or computed by PubChem | |
Retrosynthesis Analysis
AI-Powered Synthesis Planning: Our tool employs the Template_relevance Pistachio, Template_relevance Bkms_metabolic, Template_relevance Pistachio_ringbreaker, Template_relevance Reaxys, Template_relevance Reaxys_biocatalysis model, leveraging a vast database of chemical reactions to predict feasible synthetic routes.
One-Step Synthesis Focus: Specifically designed for one-step synthesis, it provides concise and direct routes for your target compounds, streamlining the synthesis process.
Accurate Predictions: Utilizing the extensive PISTACHIO, BKMS_METABOLIC, PISTACHIO_RINGBREAKER, REAXYS, REAXYS_BIOCATALYSIS database, our tool offers high-accuracy predictions, reflecting the latest in chemical research and data.
Strategy Settings
Precursor scoring | Relevance Heuristic |
---|---|
Min. plausibility | 0.01 |
Model | Template_relevance |
Template Set | Pistachio/Bkms_metabolic/Pistachio_ringbreaker/Reaxys/Reaxys_biocatalysis |
Top-N result to add to graph | 6 |
Feasible Synthetic Routes
Disclaimer and Information on In-Vitro Research Products
Please be aware that all articles and product information presented on BenchChem are intended solely for informational purposes. The products available for purchase on BenchChem are specifically designed for in-vitro studies, which are conducted outside of living organisms. In-vitro studies, derived from the Latin term "in glass," involve experiments performed in controlled laboratory settings using cells or tissues. It is important to note that these products are not categorized as medicines or drugs, and they have not received approval from the FDA for the prevention, treatment, or cure of any medical condition, ailment, or disease. We must emphasize that any form of bodily introduction of these products into humans or animals is strictly prohibited by law. It is essential to adhere to these guidelines to ensure compliance with legal and ethical standards in research and experimentation.