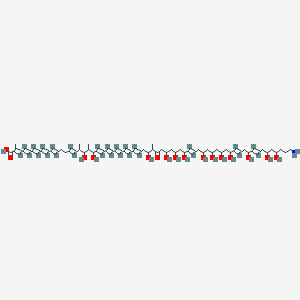
Linearmycin B
Overview
Description
Synthesis Analysis
Linearmycins are produced by Streptomyces sp. strain Mg1 and are incorporated into extracellular vesicles . The insolubility of purified linearmycins led to the hypothesis of an extracellular trafficking mechanism to deliver the lytic activity .Chemical Reactions Analysis
Linearmycins inhibit the growth of all Gram-positive bacteria tested, but lysis was limited to some Bacillus species . Linearmycin-induced lysis occurred even when cellular metabolism and growth were inhibited .Scientific Research Applications
Antibacterial Activity
Linearmycin B belongs to the linearmycin family of polyketides. Originally classified as antifungal metabolites, these compounds have demonstrated potent antibacterial properties. Linearmycins inhibit the growth of various Gram-positive bacteria, including Bacillus species. Unlike cell-wall targeting antibiotics, linearmycins directly target the cytoplasmic membrane, leading to rapid depolarization and loss of viability .
Membrane Disruption
Linearmycins are lytic membrane-targeting antibiotics. They disrupt lipid bilayers without requiring other cellular components. This unique mechanism of action makes them distinct from traditional antibiotics and highlights their potential as novel therapeutic agents .
Extracellular Vesicle Production
Recent research has revealed a connection between linearmycin biosynthesis and extracellular vesicle (EV) production. Streptomyces sp. strain Mg1, which produces linearmycins, also generates EVs. The integration of linearmycin biosynthesis with EV production suggests a deep link between specialized metabolism and bacterial membrane physiology .
Future Directions
properties
IUPAC Name |
(2E,4E,6E,8E,10E,14E,20E,22E,24E,26E,28E,40E,50E,54E)-62-amino-17,19,31,35,37,39,43,45,47,49,53,57,59-tridecahydroxy-2,16,18,32-tetramethyl-33-oxodohexaconta-2,4,6,8,10,14,20,22,24,26,28,40,50,54-tetradecaenoic acid | |
---|---|---|
Details | Computed by LexiChem 2.6.6 (PubChem release 2019.06.18) | |
Source | PubChem | |
URL | https://pubchem.ncbi.nlm.nih.gov | |
Description | Data deposited in or computed by PubChem | |
InChI |
InChI=1S/C66H103NO16/c1-48(29-21-17-13-9-6-5-7-10-14-18-22-30-49(2)66(82)83)65(81)51(4)63(79)39-24-20-16-12-8-11-15-19-23-38-62(78)50(3)64(80)47-61(77)46-60(76)44-56(72)36-27-35-55(71)43-59(75)45-58(74)42-54(70)34-26-32-52(68)31-25-33-53(69)41-57(73)37-28-40-67/h5-12,14-16,18-27,29-31,34,36,39,48,50-63,65,68-79,81H,13,17,28,32-33,35,37-38,40-47,67H2,1-4H3,(H,82,83)/b7-5+,9-6+,12-8+,14-10+,15-11+,20-16+,22-18+,23-19+,29-21+,31-25+,34-26+,36-27+,39-24+,49-30+ | |
Details | Computed by InChI 1.0.5 (PubChem release 2019.06.18) | |
Source | PubChem | |
URL | https://pubchem.ncbi.nlm.nih.gov | |
Description | Data deposited in or computed by PubChem | |
InChI Key |
DHFLEVLIHWJIAE-OTPULODUSA-N | |
Details | Computed by InChI 1.0.5 (PubChem release 2019.06.18) | |
Source | PubChem | |
URL | https://pubchem.ncbi.nlm.nih.gov | |
Description | Data deposited in or computed by PubChem | |
Canonical SMILES |
CC(C=CCCC=CC=CC=CC=CC=C(C)C(=O)O)C(C(C)C(C=CC=CC=CC=CC=CCC(C(C)C(=O)CC(CC(CC(C=CCC(CC(CC(CC(C=CCC(C=CCC(CC(CCCN)O)O)O)O)O)O)O)O)O)O)O)O)O | |
Details | Computed by OEChem 2.1.5 (PubChem release 2019.06.18) | |
Source | PubChem | |
URL | https://pubchem.ncbi.nlm.nih.gov | |
Description | Data deposited in or computed by PubChem | |
Isomeric SMILES |
CC(/C=C/CC/C=C/C=C/C=C/C=C/C=C(\C)/C(=O)O)C(C(C)C(/C=C/C=C/C=C/C=C/C=C/CC(C(C)C(=O)CC(CC(CC(/C=C/CC(CC(CC(CC(/C=C/CC(/C=C/CC(CC(CCCN)O)O)O)O)O)O)O)O)O)O)O)O)O | |
Details | Computed by OEChem 2.1.5 (PubChem release 2019.06.18) | |
Source | PubChem | |
URL | https://pubchem.ncbi.nlm.nih.gov | |
Description | Data deposited in or computed by PubChem | |
Molecular Formula |
C66H103NO16 | |
Details | Computed by PubChem 2.1 (PubChem release 2019.06.18) | |
Source | PubChem | |
URL | https://pubchem.ncbi.nlm.nih.gov | |
Description | Data deposited in or computed by PubChem | |
Molecular Weight |
1166.5 g/mol | |
Details | Computed by PubChem 2.1 (PubChem release 2021.05.07) | |
Source | PubChem | |
URL | https://pubchem.ncbi.nlm.nih.gov | |
Description | Data deposited in or computed by PubChem | |
Product Name |
Linearmycin B |
Retrosynthesis Analysis
AI-Powered Synthesis Planning: Our tool employs the Template_relevance Pistachio, Template_relevance Bkms_metabolic, Template_relevance Pistachio_ringbreaker, Template_relevance Reaxys, Template_relevance Reaxys_biocatalysis model, leveraging a vast database of chemical reactions to predict feasible synthetic routes.
One-Step Synthesis Focus: Specifically designed for one-step synthesis, it provides concise and direct routes for your target compounds, streamlining the synthesis process.
Accurate Predictions: Utilizing the extensive PISTACHIO, BKMS_METABOLIC, PISTACHIO_RINGBREAKER, REAXYS, REAXYS_BIOCATALYSIS database, our tool offers high-accuracy predictions, reflecting the latest in chemical research and data.
Strategy Settings
Precursor scoring | Relevance Heuristic |
---|---|
Min. plausibility | 0.01 |
Model | Template_relevance |
Template Set | Pistachio/Bkms_metabolic/Pistachio_ringbreaker/Reaxys/Reaxys_biocatalysis |
Top-N result to add to graph | 6 |
Feasible Synthetic Routes
Disclaimer and Information on In-Vitro Research Products
Please be aware that all articles and product information presented on BenchChem are intended solely for informational purposes. The products available for purchase on BenchChem are specifically designed for in-vitro studies, which are conducted outside of living organisms. In-vitro studies, derived from the Latin term "in glass," involve experiments performed in controlled laboratory settings using cells or tissues. It is important to note that these products are not categorized as medicines or drugs, and they have not received approval from the FDA for the prevention, treatment, or cure of any medical condition, ailment, or disease. We must emphasize that any form of bodily introduction of these products into humans or animals is strictly prohibited by law. It is essential to adhere to these guidelines to ensure compliance with legal and ethical standards in research and experimentation.