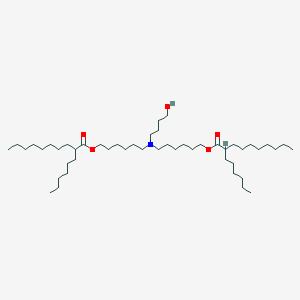
ALC-0315
Overview
Description
Mechanism of Action
Target of Action
ALC-0315, also known as ((4-Hydroxybutyl)azanediyl)bis(hexane-6,1-diyl)bis(2-hexyldecanoate), is a synthetic lipid . Its primary target is the messenger RNA (mRNA) that carries the genetic information for the SARS-CoV-2 spike protein formation in the human body .
Mode of Action
This compound forms lipid nanoparticles (LNPs) that encapsulate and protect the otherwise fragile mRNA . These nanoparticles promote the uptake of therapeutically effective nucleic acids such as oligonucleotides or mRNA both in vitro and in vivo . Below physiological pH, this compound becomes protonated at the nitrogen atom, yielding an ammonium cation that is attracted to the mRNA, which is anionic .
Biochemical Pathways
When this compound interacts with the acidic endosome, the tertiary amine becomes protonated and forms cone-shaped ion pairs that drive the transition from a bilayer to an inverted hexagon phase . This phase promotes endosomal escape and the release of the mRNA into the cytosol .
Pharmacokinetics
It is known that this compound plays a crucial role in the function of lnps by stabilizing the negatively charged mrna . The nature and site of chemical alterations in this compound resulting from forced degradation have been elucidated using structurally diagnostic fragment ions produced by electron-activated dissociation (EAD) .
Result of Action
Once the lipid nanoparticle which encapsulates the mRNA has been absorbed into antigen-presenting cells (a process called receptor-mediated endocytosis), the more acidic environment within the endosome fully protonates the this compound. As a result, the nanoparticle releases its payload of mRNA . This mRNA then triggers the body to naturally produce antibodies and stimulates immune cells to protect against COVID-19 .
Action Environment
The action of this compound is influenced by environmental factors such as pH. The acidic environment within the endosome is crucial for the full protonation of this compound and the subsequent release of mRNA . Furthermore, the effectiveness of this compound can be attenuated by lipid impurities in the LNP .
Biochemical Analysis
Biochemical Properties
Lipid ALC-0315 plays a crucial role in incorporating and protecting the negatively charged RNA payload, facilitating its penetration and release . Below physiological pH, Lipid this compound becomes protonated at the nitrogen atom, yielding an ammonium cation that is attracted to the messenger RNA (mRNA), which is anionic .
Cellular Effects
Lipid this compound is a key component of the lipid nanoparticles used to encapsulate the mRNA in the Biontech–Pfizer COVID-19 vaccine . These nanoparticles promote the uptake of therapeutically effective nucleic acids such as oligonucleotides or mRNA both in vitro and in vivo . When Lipid this compound interacts with the acidic endosome, the tertiary amine becomes protonated and forms cone-shaped ion pairs that drive the transition from a bilayer to an inverted hexagon phase. This phase promotes endosomal escape and the release of the mRNA into the cytosol .
Molecular Mechanism
The chemical properties of Lipid this compound as a tertiary amine mean that its cation can form an ionic bond to the messenger RNA which carries the genetic information for the SARS-CoV-2 spike protein formation in the human body . Once the lipid nanoparticle which encapsulates the mRNA has been absorbed into antigen-presenting cells (a process called receptor-mediated endocytosis) the more acidic environment within the endosome fully protonates the Lipid this compound. As a result, the nanoparticle releases its payload of mRNA .
Temporal Effects in Laboratory Settings
Lipid this compound was found to be stable after an approximately 4-hour incubation with hepatocytes from various species . This suggests that Lipid this compound has good stability in biological systems, which is crucial for its role in mRNA delivery.
Dosage Effects in Animal Models
The toxicity of the Lipid this compound was assessed in one species as part of the repeat dose study with the vaccine
Metabolic Pathways
In vitro and in vivo studies indicated Lipid this compound is metabolized slowly by hydrolytic metabolism of the ester and amide functionalities, respectively, across the species evaluated .
Transport and Distribution
Lipid this compound is an ionizable cationic lipid used in combination with other lipids to form lipid nanoparticles (LNPs). Cationic lipids are used to complex negatively charged nucleic acid cargo and make up the center of an LNP delivery system .
Subcellular Localization
Given its role in forming lipid nanoparticles for mRNA delivery, it is likely to be found in the cytoplasm after endocytosis, where it facilitates the release of mRNA .
Preparation Methods
The preparation of ALC-0315 was first described in a patent application by Acuitas Therapeutics in 2017 . The synthetic route involves a reductive amination reaction in which 4-aminobutanol is condensed with a lipid aldehyde, using sodium triacetoxyborohydride as the reducing agent to convert the intermediate imines to the amine of the product . The reaction can be summarized as follows:
2(C8H17)(C6H13)CHCO2(CH2)5CHO+H2N(CH2)4OH+2NaBH(O2CCH3)3→ALC−0315
Another method involves azeotropically dehydrating 6-halogenated hexanol, 2-hexyldecanoic acid, and a water-carrying agent to perform an esterification reaction, followed by an amination reaction with 4-aminobutanol in the presence of an acid-binding agent .
Chemical Reactions Analysis
ALC-0315 undergoes various chemical reactions, including:
Scientific Research Applications
ALC-0315 is primarily used in the formulation of lipid nanoparticles for mRNA delivery. These nanoparticles are crucial for the stability and delivery of mRNA in vaccines, such as the Pfizer-BioNTech COVID-19 vaccine . The lipid nanoparticles protect the mRNA from degradation and facilitate its uptake by cells, promoting the expression of the encoded protein . Additionally, this compound is used in research on nucleic acid therapeutics, including small interfering RNA (siRNA) and oligonucleotides .
Comparison with Similar Compounds
ALC-0315 is compared with other ionizable cationic lipids used in lipid nanoparticle formulations. Similar compounds include:
DLin-MC3-DMA: A cationic lipid used in lipid nanoparticle formulations for siRNA delivery.
SM-102: An ionizable lipid used in the Moderna COVID-19 vaccine.
This compound is unique due to its specific structure, which allows for efficient encapsulation and delivery of mRNA, as well as its role in the Pfizer-BioNTech COVID-19 vaccine .
Properties
IUPAC Name |
6-[6-(2-hexyldecanoyloxy)hexyl-(4-hydroxybutyl)amino]hexyl 2-hexyldecanoate | |
---|---|---|
Details | Computed by LexiChem 2.6.6 (PubChem release 2019.06.18) | |
Source | PubChem | |
URL | https://pubchem.ncbi.nlm.nih.gov | |
Description | Data deposited in or computed by PubChem | |
InChI |
InChI=1S/C48H95NO5/c1-5-9-13-17-19-27-37-45(35-25-15-11-7-3)47(51)53-43-33-23-21-29-39-49(41-31-32-42-50)40-30-22-24-34-44-54-48(52)46(36-26-16-12-8-4)38-28-20-18-14-10-6-2/h45-46,50H,5-44H2,1-4H3 | |
Details | Computed by InChI 1.0.5 (PubChem release 2019.06.18) | |
Source | PubChem | |
URL | https://pubchem.ncbi.nlm.nih.gov | |
Description | Data deposited in or computed by PubChem | |
InChI Key |
QGWBEETXHOVFQS-UHFFFAOYSA-N | |
Details | Computed by InChI 1.0.5 (PubChem release 2019.06.18) | |
Source | PubChem | |
URL | https://pubchem.ncbi.nlm.nih.gov | |
Description | Data deposited in or computed by PubChem | |
Canonical SMILES |
CCCCCCCCC(CCCCCC)C(=O)OCCCCCCN(CCCCCCOC(=O)C(CCCCCC)CCCCCCCC)CCCCO | |
Details | Computed by OEChem 2.1.5 (PubChem release 2019.06.18) | |
Source | PubChem | |
URL | https://pubchem.ncbi.nlm.nih.gov | |
Description | Data deposited in or computed by PubChem | |
Molecular Formula |
C48H95NO5 | |
Details | Computed by PubChem 2.1 (PubChem release 2019.06.18) | |
Source | PubChem | |
URL | https://pubchem.ncbi.nlm.nih.gov | |
Description | Data deposited in or computed by PubChem | |
DSSTOX Substance ID |
DTXSID701336663 | |
Record name | ALC-0315 | |
Source | EPA DSSTox | |
URL | https://comptox.epa.gov/dashboard/DTXSID701336663 | |
Description | DSSTox provides a high quality public chemistry resource for supporting improved predictive toxicology. | |
Molecular Weight |
766.3 g/mol | |
Details | Computed by PubChem 2.1 (PubChem release 2021.05.07) | |
Source | PubChem | |
URL | https://pubchem.ncbi.nlm.nih.gov | |
Description | Data deposited in or computed by PubChem | |
CAS No. |
2036272-55-4 | |
Record name | ALC-0315 | |
Source | ChemIDplus | |
URL | https://pubchem.ncbi.nlm.nih.gov/substance/?source=chemidplus&sourceid=2036272554 | |
Description | ChemIDplus is a free, web search system that provides access to the structure and nomenclature authority files used for the identification of chemical substances cited in National Library of Medicine (NLM) databases, including the TOXNET system. | |
Record name | ALC-0315 | |
Source | EPA DSSTox | |
URL | https://comptox.epa.gov/dashboard/DTXSID701336663 | |
Description | DSSTox provides a high quality public chemistry resource for supporting improved predictive toxicology. | |
Record name | ((4-HYDROXYBUTYL)AZANEDIYL)BIS(HEXANE-6,1-DIYL)BIS(2-HEXYLDECANOATE) | |
Source | FDA Global Substance Registration System (GSRS) | |
URL | https://gsrs.ncats.nih.gov/ginas/app/beta/substances/AVX8DX713V | |
Description | The FDA Global Substance Registration System (GSRS) enables the efficient and accurate exchange of information on what substances are in regulated products. Instead of relying on names, which vary across regulatory domains, countries, and regions, the GSRS knowledge base makes it possible for substances to be defined by standardized, scientific descriptions. | |
Explanation | Unless otherwise noted, the contents of the FDA website (www.fda.gov), both text and graphics, are not copyrighted. They are in the public domain and may be republished, reprinted and otherwise used freely by anyone without the need to obtain permission from FDA. Credit to the U.S. Food and Drug Administration as the source is appreciated but not required. | |
Retrosynthesis Analysis
AI-Powered Synthesis Planning: Our tool employs the Template_relevance Pistachio, Template_relevance Bkms_metabolic, Template_relevance Pistachio_ringbreaker, Template_relevance Reaxys, Template_relevance Reaxys_biocatalysis model, leveraging a vast database of chemical reactions to predict feasible synthetic routes.
One-Step Synthesis Focus: Specifically designed for one-step synthesis, it provides concise and direct routes for your target compounds, streamlining the synthesis process.
Accurate Predictions: Utilizing the extensive PISTACHIO, BKMS_METABOLIC, PISTACHIO_RINGBREAKER, REAXYS, REAXYS_BIOCATALYSIS database, our tool offers high-accuracy predictions, reflecting the latest in chemical research and data.
Strategy Settings
Precursor scoring | Relevance Heuristic |
---|---|
Min. plausibility | 0.01 |
Model | Template_relevance |
Template Set | Pistachio/Bkms_metabolic/Pistachio_ringbreaker/Reaxys/Reaxys_biocatalysis |
Top-N result to add to graph | 6 |
Feasible Synthetic Routes
Disclaimer and Information on In-Vitro Research Products
Please be aware that all articles and product information presented on BenchChem are intended solely for informational purposes. The products available for purchase on BenchChem are specifically designed for in-vitro studies, which are conducted outside of living organisms. In-vitro studies, derived from the Latin term "in glass," involve experiments performed in controlled laboratory settings using cells or tissues. It is important to note that these products are not categorized as medicines or drugs, and they have not received approval from the FDA for the prevention, treatment, or cure of any medical condition, ailment, or disease. We must emphasize that any form of bodily introduction of these products into humans or animals is strictly prohibited by law. It is essential to adhere to these guidelines to ensure compliance with legal and ethical standards in research and experimentation.