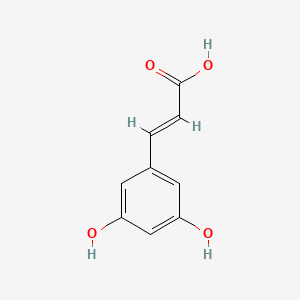
3,5-Dihydroxycinnamic acid
Overview
Description
3,5-Dihydroxycinnamic acid is a hydroxycinnamic acid, which is an isomer of caffeic acid . It is a metabolite found in human urine . This compound belongs to the class of organic compounds known as hydroxycinnamic acids .
Synthesis Analysis
Anti-inflammatory activities of ten new synthesized derivatives of this compound were tested in 12-O-tetradecanoylphorbol 13-acetate-induced mouse ear edema .Molecular Structure Analysis
The molecular formula of this compound is C9H8O4 . Cinnamic acid derivatives are generated through the shikimic acid pathway. They belong to the phenylpropanoid class . The starting materials in biosynthesis are phenyl-based amino acids, named L-Phe and L-Tyr .Chemical Reactions Analysis
Hydroxycinnamic acids can form esters with quinic acid at all four disposable hydroxyl groups . Thus, derivatives with ester group in 1-, 1,3-, 1,4-, 1,5-, 3,4-, 3,5-, and 4,5- were identified .Physical And Chemical Properties Analysis
The molar mass of this compound is 180.159 g·mol−1 . Data are given for materials in their standard state (at 25 °C [77 °F], 100 kPa) .Scientific Research Applications
Anti-Inflammatory Properties
3,5-Dihydroxycinnamic acid and its derivatives exhibit significant anti-inflammatory activities. A study synthesized ten derivatives of this compound, testing their effectiveness in reducing inflammation in mouse ear edema. One compound, in particular, demonstrated pronounced anti-inflammatory effects by reducing key pro-inflammatory markers such as tumor necrosis factor-α and interleukins, as well as decreasing activated neutrophil levels. This suggests the potential value of this compound derivatives in anti-inflammatory drug development (Zhang et al., 2015).
Antioxidant Activity
Caffeic acid, a form of 3,4-dihydroxycinnamic acid, is known for its antioxidant properties. This compound is present in various foods and demonstrates abilities like scavenging free radicals and reducing oxidative stress. Studies indicate that it can be an effective natural antioxidant in different food products (Gülçin, 2006), (Khan et al., 2016).
Photoprotection and UV Absorption
The encapsulation of organic UV ray absorbents, including 3,4-dihydroxycinnamic acid, into layered double hydroxide, has been explored for its photochemical properties. These nanohybrids, combining organic UV absorbents with layered double hydroxide, show exceptional UV ray absorption, which could be beneficial in photoprotection applications (Khan et al., 2011).
Potential in Cancer Treatment
A novel 2,5-dihydroxycinnamic acid-based compound was discovered as a potent inhibitor of 5-lipoxygenase, an enzyme involved in leukotriene biosynthesis. This compound showed significant potential in reducing the viability of renal cancer cells and induced apoptosis in these cells, highlighting its potential as an anti-cancer agent (Selka et al., 2019).
Mechanism of Action
Target of Action
3,5-Dihydroxycinnamic acid, also known as a hydroxycinnamic acid , is a natural phenolic compoundIt’s worth noting that hydroxycinnamic acids are known to interact with various biological targets, influencing numerous physiological processes .
Mode of Action
It’s known that hydroxycinnamic acids can interact with their targets in various ways, leading to different biological effects . For instance, they can act as antioxidants, neutralizing harmful free radicals in the body .
Biochemical Pathways
This compound is derived from the plant phenylpropanoid pathway . This pathway is responsible for the production of a wide range of compounds that play crucial roles in plant growth and development. The compound is a metabolite found in human urine .
Pharmacokinetics
It’s known that hydroxycinnamic acids, in general, can be absorbed and metabolized by the human body .
Result of Action
Hydroxycinnamic acids are known to exhibit various biological activities, including antioxidant, anti-inflammatory, and antibacterial effects . For instance, one derivative of this compound showed a pronounced anti-inflammatory effect by reducing mRNA and protein synthesis of tumor necrosis factor-α, interleukins 1β and 6, and also by decreasing the levels of activated neutrophil infiltrates .
Action Environment
The action, efficacy, and stability of this compound can be influenced by various environmental factors. For instance, the compound’s action can be affected by the presence of other compounds in the environment, the pH of the environment, and the temperature .
Safety and Hazards
Future Directions
Hydroxycinnamic acids and derivatives have been investigated and applied in several research fields for their anti-oxidant, anti-inflammatory, and anti-bacterial activities . They have potential roles as valid alternatives to current therapies, supporting the management and rebalancing of skin disorders and diseases at different levels .
Biochemical Analysis
Biochemical Properties
It is known that it is a hydroxycinnamic acid, which is a class of compounds containing a cinnamic acid where the benzene ring is hydroxylated .
Cellular Effects
This derivative acted by reducing mRNA and protein synthesis of tumor necrosis factor-α, interleukins 1β and 6, and also by decreasing the levels of activated neutrophil infiltrates .
Molecular Mechanism
A derivative of this compound has been shown to inhibit the production of cyclooxygenase-2-catalyzed prostaglandin E2 from lipopolysaccharide-treated RAW 264.7 cells, and also inhibited 5-lipoxygenase production from A23187-treated RBL-1 cells, consequently reducing leukotriene B4 production .
Metabolic Pathways
BioTransformer predicts that 3,5-Dihydroxycinnamic acid is a product of 3, 4, 5- trihydroxycinnamic acid metabolism via a -4p-dehydroxylation-of-substituted-benzene reaction occurring in human gut microbiota and catalyzed by a dehydroxylase enzyme .
Properties
IUPAC Name |
(E)-3-(3,5-dihydroxyphenyl)prop-2-enoic acid | |
---|---|---|
Source | PubChem | |
URL | https://pubchem.ncbi.nlm.nih.gov | |
Description | Data deposited in or computed by PubChem | |
InChI |
InChI=1S/C9H8O4/c10-7-3-6(1-2-9(12)13)4-8(11)5-7/h1-5,10-11H,(H,12,13)/b2-1+ | |
Source | PubChem | |
URL | https://pubchem.ncbi.nlm.nih.gov | |
Description | Data deposited in or computed by PubChem | |
InChI Key |
MFFCZSWTQMCKFP-OWOJBTEDSA-N | |
Source | PubChem | |
URL | https://pubchem.ncbi.nlm.nih.gov | |
Description | Data deposited in or computed by PubChem | |
Canonical SMILES |
C1=C(C=C(C=C1O)O)C=CC(=O)O | |
Source | PubChem | |
URL | https://pubchem.ncbi.nlm.nih.gov | |
Description | Data deposited in or computed by PubChem | |
Isomeric SMILES |
C1=C(C=C(C=C1O)O)/C=C/C(=O)O | |
Source | PubChem | |
URL | https://pubchem.ncbi.nlm.nih.gov | |
Description | Data deposited in or computed by PubChem | |
Molecular Formula |
C9H8O4 | |
Source | PubChem | |
URL | https://pubchem.ncbi.nlm.nih.gov | |
Description | Data deposited in or computed by PubChem | |
DSSTOX Substance ID |
DTXSID301030504 | |
Record name | 3,5-Dihydroxycinnamic acid | |
Source | EPA DSSTox | |
URL | https://comptox.epa.gov/dashboard/DTXSID301030504 | |
Description | DSSTox provides a high quality public chemistry resource for supporting improved predictive toxicology. | |
Molecular Weight |
180.16 g/mol | |
Source | PubChem | |
URL | https://pubchem.ncbi.nlm.nih.gov | |
Description | Data deposited in or computed by PubChem | |
Physical Description |
Solid | |
Record name | 3,5-Dihydroxycinnamic acid | |
Source | Human Metabolome Database (HMDB) | |
URL | http://www.hmdb.ca/metabolites/HMDB0032131 | |
Description | The Human Metabolome Database (HMDB) is a freely available electronic database containing detailed information about small molecule metabolites found in the human body. | |
Explanation | HMDB is offered to the public as a freely available resource. Use and re-distribution of the data, in whole or in part, for commercial purposes requires explicit permission of the authors and explicit acknowledgment of the source material (HMDB) and the original publication (see the HMDB citing page). We ask that users who download significant portions of the database cite the HMDB paper in any resulting publications. | |
CAS No. |
127791-54-2, 28374-93-8 | |
Record name | (2E)-3-(3,5-Dihydroxyphenyl)-2-propenoic acid | |
Source | CAS Common Chemistry | |
URL | https://commonchemistry.cas.org/detail?cas_rn=127791-54-2 | |
Description | CAS Common Chemistry is an open community resource for accessing chemical information. Nearly 500,000 chemical substances from CAS REGISTRY cover areas of community interest, including common and frequently regulated chemicals, and those relevant to high school and undergraduate chemistry classes. This chemical information, curated by our expert scientists, is provided in alignment with our mission as a division of the American Chemical Society. | |
Explanation | The data from CAS Common Chemistry is provided under a CC-BY-NC 4.0 license, unless otherwise stated. | |
Record name | 3,5-Dihydroxycinnamic acid | |
Source | ChemIDplus | |
URL | https://pubchem.ncbi.nlm.nih.gov/substance/?source=chemidplus&sourceid=0028374938 | |
Description | ChemIDplus is a free, web search system that provides access to the structure and nomenclature authority files used for the identification of chemical substances cited in National Library of Medicine (NLM) databases, including the TOXNET system. | |
Record name | 3,5-Dihydroxycinnamic acid | |
Source | ChemIDplus | |
URL | https://pubchem.ncbi.nlm.nih.gov/substance/?source=chemidplus&sourceid=0127791542 | |
Description | ChemIDplus is a free, web search system that provides access to the structure and nomenclature authority files used for the identification of chemical substances cited in National Library of Medicine (NLM) databases, including the TOXNET system. | |
Record name | 3,5-Dihydroxycinnamic acid | |
Source | EPA DSSTox | |
URL | https://comptox.epa.gov/dashboard/DTXSID301030504 | |
Description | DSSTox provides a high quality public chemistry resource for supporting improved predictive toxicology. | |
Record name | 3,5-DIHYDROXYCINNAMIC ACID | |
Source | FDA Global Substance Registration System (GSRS) | |
URL | https://gsrs.ncats.nih.gov/ginas/app/beta/substances/9PD8QLS6G8 | |
Description | The FDA Global Substance Registration System (GSRS) enables the efficient and accurate exchange of information on what substances are in regulated products. Instead of relying on names, which vary across regulatory domains, countries, and regions, the GSRS knowledge base makes it possible for substances to be defined by standardized, scientific descriptions. | |
Explanation | Unless otherwise noted, the contents of the FDA website (www.fda.gov), both text and graphics, are not copyrighted. They are in the public domain and may be republished, reprinted and otherwise used freely by anyone without the need to obtain permission from FDA. Credit to the U.S. Food and Drug Administration as the source is appreciated but not required. | |
Record name | 3,5-Dihydroxycinnamic acid | |
Source | Human Metabolome Database (HMDB) | |
URL | http://www.hmdb.ca/metabolites/HMDB0032131 | |
Description | The Human Metabolome Database (HMDB) is a freely available electronic database containing detailed information about small molecule metabolites found in the human body. | |
Explanation | HMDB is offered to the public as a freely available resource. Use and re-distribution of the data, in whole or in part, for commercial purposes requires explicit permission of the authors and explicit acknowledgment of the source material (HMDB) and the original publication (see the HMDB citing page). We ask that users who download significant portions of the database cite the HMDB paper in any resulting publications. | |
Melting Point |
245 - 246 °C | |
Record name | 3,5-Dihydroxycinnamic acid | |
Source | Human Metabolome Database (HMDB) | |
URL | http://www.hmdb.ca/metabolites/HMDB0032131 | |
Description | The Human Metabolome Database (HMDB) is a freely available electronic database containing detailed information about small molecule metabolites found in the human body. | |
Explanation | HMDB is offered to the public as a freely available resource. Use and re-distribution of the data, in whole or in part, for commercial purposes requires explicit permission of the authors and explicit acknowledgment of the source material (HMDB) and the original publication (see the HMDB citing page). We ask that users who download significant portions of the database cite the HMDB paper in any resulting publications. | |
Retrosynthesis Analysis
AI-Powered Synthesis Planning: Our tool employs the Template_relevance Pistachio, Template_relevance Bkms_metabolic, Template_relevance Pistachio_ringbreaker, Template_relevance Reaxys, Template_relevance Reaxys_biocatalysis model, leveraging a vast database of chemical reactions to predict feasible synthetic routes.
One-Step Synthesis Focus: Specifically designed for one-step synthesis, it provides concise and direct routes for your target compounds, streamlining the synthesis process.
Accurate Predictions: Utilizing the extensive PISTACHIO, BKMS_METABOLIC, PISTACHIO_RINGBREAKER, REAXYS, REAXYS_BIOCATALYSIS database, our tool offers high-accuracy predictions, reflecting the latest in chemical research and data.
Strategy Settings
Precursor scoring | Relevance Heuristic |
---|---|
Min. plausibility | 0.01 |
Model | Template_relevance |
Template Set | Pistachio/Bkms_metabolic/Pistachio_ringbreaker/Reaxys/Reaxys_biocatalysis |
Top-N result to add to graph | 6 |
Feasible Synthetic Routes
Q1: What are the key biological activities reported for 3,5-dihydroxycinnamic acid derivatives?
A1: Research suggests that this compound derivatives exhibit potent anti-inflammatory activities. [] A study highlighted that these derivatives, particularly compound 7 (a specific derivative detailed in the study), demonstrated superior anti-inflammatory effects compared to 3,4-dihydroxycinnamic acid. [] This makes this compound derivatives, especially compound 7, promising candidates for anti-inflammatory drug development. []
Q2: How do this compound derivatives exert their anti-inflammatory effects?
A2: Studies reveal a multifaceted mechanism of action for the anti-inflammatory properties of this compound derivatives. [] They have been shown to:
- Reduce inflammation markers: Compound 7, a derivative, effectively decreased both the mRNA and protein levels of key inflammatory cytokines like tumor necrosis factor-α and interleukins 1β and 6. []
- Inhibit inflammatory cell infiltration: Compound 7 significantly diminished the infiltration of activated neutrophils into inflamed tissues. []
- Suppress arachidonic acid pathway: Compound 7 demonstrated substantial suppression of edema induced by arachidonic acid. []
- Target cyclooxygenase-2 (COX-2) and 5-lipoxygenase (5-LOX) enzymes: Cell-based assays showed that compound 7 inhibited COX-2 activity in lipopolysaccharide-stimulated RAW 264.7 cells, thereby reducing prostaglandin E2 production. [] Additionally, it inhibited 5-LOX activity in A23187-treated RBL-1 cells, leading to decreased leukotriene B4 production. []
Q3: What other natural sources, besides whole grains, contain this compound?
A4: this compound has been identified in the fruits of Acanthopanax sessiliflorus Seeman (family Araliaceae). [] This marks the first reported instance of this compound being isolated from this particular plant species. []
Q4: Have any analytical methods been developed to quantify this compound and its metabolites?
A4: Scientists have been exploring both chromatographic and immunoassay techniques for analyzing this compound and its metabolites:
- Chromatographic methods: Gas chromatography-mass spectrometry (GC-MS) and liquid chromatography-tandem mass spectrometry (LC-MS/MS) have been employed for the identification and quantification of these compounds. [, ]
Q5: Does the chemical structure of this compound relate to its activity?
A6: Structural features of this compound derivatives play a crucial role in their antioxidant and antiproliferative properties. [] Research on caffeic acid phenethyl ester (CAPE) derivatives reveals that:
Disclaimer and Information on In-Vitro Research Products
Please be aware that all articles and product information presented on BenchChem are intended solely for informational purposes. The products available for purchase on BenchChem are specifically designed for in-vitro studies, which are conducted outside of living organisms. In-vitro studies, derived from the Latin term "in glass," involve experiments performed in controlled laboratory settings using cells or tissues. It is important to note that these products are not categorized as medicines or drugs, and they have not received approval from the FDA for the prevention, treatment, or cure of any medical condition, ailment, or disease. We must emphasize that any form of bodily introduction of these products into humans or animals is strictly prohibited by law. It is essential to adhere to these guidelines to ensure compliance with legal and ethical standards in research and experimentation.