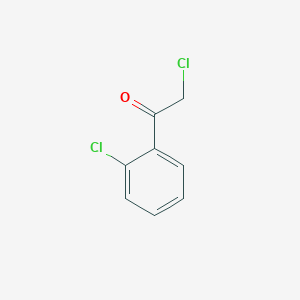
2-Chloro-1-(2-chlorophenyl)ethanone
Overview
Description
2-Chloro-1-(2-chlorophenyl)ethanone is an organic compound with the molecular formula C8H6Cl2O. It is a chlorinated derivative of acetophenone and is known for its use in various chemical reactions and applications. This compound is characterized by the presence of two chlorine atoms attached to the phenyl ring and the ethanone group.
Preparation Methods
Synthetic Routes and Reaction Conditions
2-Chloro-1-(2-chlorophenyl)ethanone can be synthesized through several methods. One common method involves the chlorination of 2-chloroacetophenone. The reaction typically takes place in the presence of a chlorinating agent such as thionyl chloride or phosphorus pentachloride. The reaction conditions often include a solvent like dichloromethane and a temperature range of 0-25°C to ensure controlled chlorination.
Industrial Production Methods
In industrial settings, the production of this compound may involve continuous flow processes to ensure high yield and purity. The use of automated systems and reactors allows for precise control over reaction parameters, leading to efficient production of the compound.
Chemical Reactions Analysis
Types of Reactions
2-Chloro-1-(2-chlorophenyl)ethanone undergoes various chemical reactions, including:
Nucleophilic Substitution: The chlorine atoms on the phenyl ring can be substituted by nucleophiles such as amines, thiols, or alkoxides.
Reduction: The carbonyl group can be reduced to an alcohol using reducing agents like sodium borohydride or lithium aluminum hydride.
Oxidation: The compound can be oxidized to form carboxylic acids or other oxidized derivatives using oxidizing agents like potassium permanganate or chromium trioxide.
Common Reagents and Conditions
Nucleophilic Substitution: Reagents such as sodium amide or potassium thiolate in solvents like ethanol or dimethyl sulfoxide.
Reduction: Sodium borohydride in methanol or lithium aluminum hydride in ether.
Oxidation: Potassium permanganate in aqueous solution or chromium trioxide in acetic acid.
Major Products Formed
Nucleophilic Substitution: Substituted phenyl ethanones.
Reduction: 2-Chloro-1-(2-chlorophenyl)ethanol.
Oxidation: 2-Chloro-1-(2-chlorophenyl)acetic acid.
Scientific Research Applications
2-Chloro-1-(2-chlorophenyl)ethanone has several applications in scientific research:
Chemistry: Used as an intermediate in the synthesis of various organic compounds, including pharmaceuticals and agrochemicals.
Biology: Employed in the study of enzyme-catalyzed reactions and as a probe for investigating biochemical pathways.
Medicine: Investigated for its potential use in drug development and as a precursor for active pharmaceutical ingredients.
Industry: Utilized in the production of specialty chemicals and as a reagent in chemical manufacturing processes.
Mechanism of Action
The mechanism of action of 2-chloro-1-(2-chlorophenyl)ethanone involves its interaction with specific molecular targets. The compound can act as an electrophile, reacting with nucleophilic sites on biomolecules such as proteins and nucleic acids. This interaction can lead to the formation of covalent bonds, altering the function of the target molecules and affecting various biochemical pathways.
Comparison with Similar Compounds
Similar Compounds
- 2-Chloro-1-(3-chlorophenyl)ethanone
- 2-Chloro-1-(4-chlorophenyl)ethanone
- 2-Chloroacetophenone
Uniqueness
2-Chloro-1-(2-chlorophenyl)ethanone is unique due to the specific positioning of the chlorine atoms on the phenyl ring, which influences its reactivity and interaction with other molecules. This positional isomerism can result in different chemical and biological properties compared to its analogs.
Properties
IUPAC Name |
2-chloro-1-(2-chlorophenyl)ethanone | |
---|---|---|
Details | Computed by LexiChem 2.6.6 (PubChem release 2019.06.18) | |
Source | PubChem | |
URL | https://pubchem.ncbi.nlm.nih.gov | |
Description | Data deposited in or computed by PubChem | |
InChI |
InChI=1S/C8H6Cl2O/c9-5-8(11)6-3-1-2-4-7(6)10/h1-4H,5H2 | |
Details | Computed by InChI 1.0.5 (PubChem release 2019.06.18) | |
Source | PubChem | |
URL | https://pubchem.ncbi.nlm.nih.gov | |
Description | Data deposited in or computed by PubChem | |
InChI Key |
SOGLGKRIVMZMSK-UHFFFAOYSA-N | |
Details | Computed by InChI 1.0.5 (PubChem release 2019.06.18) | |
Source | PubChem | |
URL | https://pubchem.ncbi.nlm.nih.gov | |
Description | Data deposited in or computed by PubChem | |
Canonical SMILES |
C1=CC=C(C(=C1)C(=O)CCl)Cl | |
Details | Computed by OEChem 2.1.5 (PubChem release 2019.06.18) | |
Source | PubChem | |
URL | https://pubchem.ncbi.nlm.nih.gov | |
Description | Data deposited in or computed by PubChem | |
Molecular Formula |
C8H6Cl2O | |
Details | Computed by PubChem 2.1 (PubChem release 2019.06.18) | |
Source | PubChem | |
URL | https://pubchem.ncbi.nlm.nih.gov | |
Description | Data deposited in or computed by PubChem | |
DSSTOX Substance ID |
DTXSID80565086 | |
Record name | 2-Chloro-1-(2-chlorophenyl)ethan-1-one | |
Source | EPA DSSTox | |
URL | https://comptox.epa.gov/dashboard/DTXSID80565086 | |
Description | DSSTox provides a high quality public chemistry resource for supporting improved predictive toxicology. | |
Molecular Weight |
189.04 g/mol | |
Details | Computed by PubChem 2.1 (PubChem release 2021.05.07) | |
Source | PubChem | |
URL | https://pubchem.ncbi.nlm.nih.gov | |
Description | Data deposited in or computed by PubChem | |
CAS No. |
4209-25-0 | |
Record name | 2-Chloro-1-(2-chlorophenyl)ethan-1-one | |
Source | EPA DSSTox | |
URL | https://comptox.epa.gov/dashboard/DTXSID80565086 | |
Description | DSSTox provides a high quality public chemistry resource for supporting improved predictive toxicology. | |
Record name | 4209-25-0 | |
Source | European Chemicals Agency (ECHA) | |
URL | https://echa.europa.eu/information-on-chemicals | |
Description | The European Chemicals Agency (ECHA) is an agency of the European Union which is the driving force among regulatory authorities in implementing the EU's groundbreaking chemicals legislation for the benefit of human health and the environment as well as for innovation and competitiveness. | |
Explanation | Use of the information, documents and data from the ECHA website is subject to the terms and conditions of this Legal Notice, and subject to other binding limitations provided for under applicable law, the information, documents and data made available on the ECHA website may be reproduced, distributed and/or used, totally or in part, for non-commercial purposes provided that ECHA is acknowledged as the source: "Source: European Chemicals Agency, http://echa.europa.eu/". Such acknowledgement must be included in each copy of the material. ECHA permits and encourages organisations and individuals to create links to the ECHA website under the following cumulative conditions: Links can only be made to webpages that provide a link to the Legal Notice page. | |
Retrosynthesis Analysis
AI-Powered Synthesis Planning: Our tool employs the Template_relevance Pistachio, Template_relevance Bkms_metabolic, Template_relevance Pistachio_ringbreaker, Template_relevance Reaxys, Template_relevance Reaxys_biocatalysis model, leveraging a vast database of chemical reactions to predict feasible synthetic routes.
One-Step Synthesis Focus: Specifically designed for one-step synthesis, it provides concise and direct routes for your target compounds, streamlining the synthesis process.
Accurate Predictions: Utilizing the extensive PISTACHIO, BKMS_METABOLIC, PISTACHIO_RINGBREAKER, REAXYS, REAXYS_BIOCATALYSIS database, our tool offers high-accuracy predictions, reflecting the latest in chemical research and data.
Strategy Settings
Precursor scoring | Relevance Heuristic |
---|---|
Min. plausibility | 0.01 |
Model | Template_relevance |
Template Set | Pistachio/Bkms_metabolic/Pistachio_ringbreaker/Reaxys/Reaxys_biocatalysis |
Top-N result to add to graph | 6 |
Feasible Synthetic Routes
Disclaimer and Information on In-Vitro Research Products
Please be aware that all articles and product information presented on BenchChem are intended solely for informational purposes. The products available for purchase on BenchChem are specifically designed for in-vitro studies, which are conducted outside of living organisms. In-vitro studies, derived from the Latin term "in glass," involve experiments performed in controlled laboratory settings using cells or tissues. It is important to note that these products are not categorized as medicines or drugs, and they have not received approval from the FDA for the prevention, treatment, or cure of any medical condition, ailment, or disease. We must emphasize that any form of bodily introduction of these products into humans or animals is strictly prohibited by law. It is essential to adhere to these guidelines to ensure compliance with legal and ethical standards in research and experimentation.