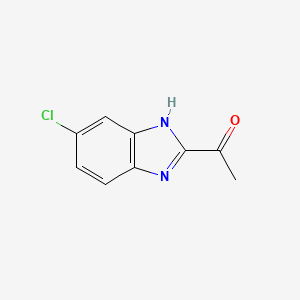
1-(6-chloro-1H-benzimidazol-2-yl)ethanone
Overview
Description
“1-(6-chloro-1H-benzimidazol-2-yl)ethanone” is a chemical compound that belongs to the class of benzimidazoles . Benzimidazoles are a broad group of compounds containing nitrogen atoms in their structure that can mimic properties of DNA bases .
Chemical Reactions Analysis
Benzimidazoles, including “1-(6-chloro-1H-benzimidazol-2-yl)ethanone”, can participate in various chemical reactions. For example, in basic medium, the benzimidazole ring (NH) proton is deprotonated forming neutral bis-chelates .Scientific Research Applications
Antiparasitic Activity
1-(6-chloro-1H-benzimidazol-2-yl)ethanone: has demonstrated promising antiparasitic properties. In a study, a series of new benzimidazolyl-2-hydrazones containing hydroxyl and methoxy groups were synthesized. These compounds exhibited potent anthelmintic activity against encapsulated Trichinella spiralis (T. spiralis) larvae in vitro, surpassing the effectiveness of clinically used anthelmintic drugs like albendazole and ivermectin. Notably, compounds 5b and 5d achieved 100% larval kill after 24 hours of incubation .
Antioxidant Properties
The same benzimidazolyl-2-hydrazones were also investigated for their antioxidant activity. Compounds 5b and 5d, which contain 2,3- and 3,4-dihydroxy groups, exhibited strong radical scavenging abilities against stable free radicals (DPPH and ABTS) and iron-induced oxidative damage. These findings suggest that 1-(6-chloro-1H-benzimidazol-2-yl)ethanone derivatives could serve as potential antioxidants .
Antibacterial and Antifungal Effects
Benzimidazole derivatives have been explored for their antibacterial and antifungal properties. While specific data on 1-(6-chloro-1H-benzimidazol-2-yl)ethanone is limited, related compounds in this class have shown activity against bacteria (Staphylococcus aureus, Staphylococcus epidermidis, Klebsiella pneumoniae, and Escherichia coli) and fungi (Candida albicans and Aspergillus niger) .
MCH-R1 Antagonism
In a different context, researchers synthesized 1-(1H-benzimidazol-6-yl) pyridin-2(1H)-one compounds. Among them, compound 513 exhibited prominent antagonistic properties against MCH-R1. Although this specific compound is not identical to 1-(6-chloro-1H-benzimidazol-2-yl)ethanone , it highlights the potential of benzimidazole-based molecules in modulating specific receptors .
Medicinal Chemistry Research
Benzimidazole derivatives have been extensively studied in medicinal chemistry. Their diverse pharmacophores make them attractive for drug design and development. While more research is needed on 1-(6-chloro-1H-benzimidazol-2-yl)ethanone , its structural features align with the broader trends observed in this class .
Mechanism of Action
Target of Action
It’s worth noting that benzimidazole derivatives, which this compound is a part of, have been reported to show a broad range of biological activities . They have been used in the development of new drugs due to their chemical and biological properties .
Mode of Action
Benzimidazole derivatives are known to interact with their targets in various ways, leading to different biological activities such as antibacterial, antimycobacterial, anti-inflammatory, antitumor, antidiabetic, anti-allergic, antipyretic, antiviral, antioxidant, anti-amoebic, antihelmintic, antifungal and ulcerogenic activities .
Biochemical Pathways
Benzimidazole derivatives are known to influence a variety of biochemical pathways due to their broad spectrum of biological activities .
Pharmacokinetics
Due to the polar nature of the imidazole ring, the pharmacokinetic parameters of imidazole-containing compounds should be improved to a great extent .
Result of Action
Benzimidazole derivatives are known to result in various biological activities, as mentioned earlier .
Action Environment
The solubility of imidazole-containing compounds in water and other polar solvents suggests that they may be influenced by the polarity of their environment .
properties
IUPAC Name |
1-(6-chloro-1H-benzimidazol-2-yl)ethanone | |
---|---|---|
Details | Computed by Lexichem TK 2.7.0 (PubChem release 2021.05.07) | |
Source | PubChem | |
URL | https://pubchem.ncbi.nlm.nih.gov | |
Description | Data deposited in or computed by PubChem | |
InChI |
InChI=1S/C9H7ClN2O/c1-5(13)9-11-7-3-2-6(10)4-8(7)12-9/h2-4H,1H3,(H,11,12) | |
Details | Computed by InChI 1.0.6 (PubChem release 2021.05.07) | |
Source | PubChem | |
URL | https://pubchem.ncbi.nlm.nih.gov | |
Description | Data deposited in or computed by PubChem | |
InChI Key |
WKFDOUULOOHNCG-UHFFFAOYSA-N | |
Details | Computed by InChI 1.0.6 (PubChem release 2021.05.07) | |
Source | PubChem | |
URL | https://pubchem.ncbi.nlm.nih.gov | |
Description | Data deposited in or computed by PubChem | |
Canonical SMILES |
CC(=O)C1=NC2=C(N1)C=C(C=C2)Cl | |
Details | Computed by OEChem 2.3.0 (PubChem release 2021.05.07) | |
Source | PubChem | |
URL | https://pubchem.ncbi.nlm.nih.gov | |
Description | Data deposited in or computed by PubChem | |
Molecular Formula |
C9H7ClN2O | |
Details | Computed by PubChem 2.1 (PubChem release 2021.05.07) | |
Source | PubChem | |
URL | https://pubchem.ncbi.nlm.nih.gov | |
Description | Data deposited in or computed by PubChem | |
Molecular Weight |
194.62 g/mol | |
Details | Computed by PubChem 2.1 (PubChem release 2021.05.07) | |
Source | PubChem | |
URL | https://pubchem.ncbi.nlm.nih.gov | |
Description | Data deposited in or computed by PubChem | |
Retrosynthesis Analysis
AI-Powered Synthesis Planning: Our tool employs the Template_relevance Pistachio, Template_relevance Bkms_metabolic, Template_relevance Pistachio_ringbreaker, Template_relevance Reaxys, Template_relevance Reaxys_biocatalysis model, leveraging a vast database of chemical reactions to predict feasible synthetic routes.
One-Step Synthesis Focus: Specifically designed for one-step synthesis, it provides concise and direct routes for your target compounds, streamlining the synthesis process.
Accurate Predictions: Utilizing the extensive PISTACHIO, BKMS_METABOLIC, PISTACHIO_RINGBREAKER, REAXYS, REAXYS_BIOCATALYSIS database, our tool offers high-accuracy predictions, reflecting the latest in chemical research and data.
Strategy Settings
Precursor scoring | Relevance Heuristic |
---|---|
Min. plausibility | 0.01 |
Model | Template_relevance |
Template Set | Pistachio/Bkms_metabolic/Pistachio_ringbreaker/Reaxys/Reaxys_biocatalysis |
Top-N result to add to graph | 6 |
Feasible Synthetic Routes
Disclaimer and Information on In-Vitro Research Products
Please be aware that all articles and product information presented on BenchChem are intended solely for informational purposes. The products available for purchase on BenchChem are specifically designed for in-vitro studies, which are conducted outside of living organisms. In-vitro studies, derived from the Latin term "in glass," involve experiments performed in controlled laboratory settings using cells or tissues. It is important to note that these products are not categorized as medicines or drugs, and they have not received approval from the FDA for the prevention, treatment, or cure of any medical condition, ailment, or disease. We must emphasize that any form of bodily introduction of these products into humans or animals is strictly prohibited by law. It is essential to adhere to these guidelines to ensure compliance with legal and ethical standards in research and experimentation.