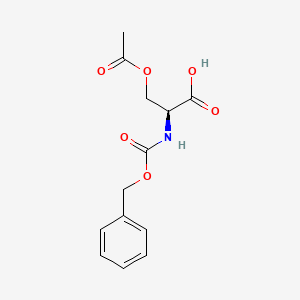
Z-O-Acetyl-L-serine
Overview
Description
Z-O-Acetyl-L-serine: is a derivative of the amino acid L-serine, where the hydroxyl group of serine is acetylated. This compound plays a significant role in the biosynthesis of cysteine in various organisms, including bacteria and plants. It is an intermediate in the metabolic pathway that converts L-serine to L-cysteine, a crucial sulfur-containing amino acid.
Mechanism of Action
Target of Action
Z-O-Acetyl-L-serine primarily targets Prostaglandin G/H synthase 1 . This enzyme plays a crucial role in the synthesis of prostanoids, a group of physiologically active lipid compounds that have diverse hormone-like effects in animals .
Mode of Action
This compound interacts with its target by converting arachidonate to prostaglandin H2 (PGH2) . This conversion is a committed step in prostanoid synthesis . The compound is involved in the constitutive production of prostanoids, particularly in the stomach and platelets .
Biochemical Pathways
The biosynthesis of cysteine from serine in bacteria, including this compound, is carried out by a two-step pathway. The first step involves the O-acetylation of serine, followed by the replacement of the acetyl group by sulfide or thiosulfate . This process is crucial for the production of sulfur-containing amino acids in the metabolic networks of various organisms .
Pharmacokinetics
It’s important to note that the absorption, distribution, metabolism, and excretion (adme) properties of a compound significantly impact its bioavailability and efficacy .
Result of Action
The action of this compound results in the production of prostanoids, particularly in the stomach and platelets . Prostanoids play a variety of roles in the body, including the regulation of inflammation and blood flow, and the formation of blood clots .
Action Environment
The action of this compound, like many other biochemical compounds, can be influenced by various environmental factors. For instance, in yeast, the metabolism and regulatory mechanisms of amino acids, including this compound, can vary under different growth environments . These factors can affect the compound’s action, efficacy, and stability.
Biochemical Analysis
Biochemical Properties
Z-O-Acetyl-L-serine plays a significant role in biochemical reactions. It provides the carbon backbone for the synthesis of cysteine . Sulfide generated through sulfate reduction is incorporated into this compound to form cysteine .
Cellular Effects
This compound influences cell function by changing the transcription levels of a specific set of genes, irrespective of the sulfur status of the plant . Treatment with external this compound increases sulfate transporter and adenosine 5′-phosphosulfate reductase gene expression .
Molecular Mechanism
The molecular mechanism of this compound involves its role as a signaling molecule. It leads to changes in transcript levels of a specific gene set irrespective of the sulfur status of the plant .
Temporal Effects in Laboratory Settings
In laboratory settings, this compound shows a certain level of stability. It is soluble in water and has a melting point of 151.5-151.8 °C .
Metabolic Pathways
This compound is involved in the metabolic pathway of sulfur assimilation, providing the carbon backbone for the synthesis of cysteine .
Preparation Methods
Synthetic Routes and Reaction Conditions:
Enzymatic Synthesis: The primary method for synthesizing Z-O-Acetyl-L-serine involves the enzyme L-serine O-acetyltransferase.
Chemical Synthesis: Another method involves the chemical acetylation of L-serine using acetic anhydride or acetyl chloride in the presence of a base such as pyridine.
Industrial Production Methods: Industrial production of this compound primarily relies on biotechnological processes involving microbial fermentation. Genetically engineered strains of bacteria such as Escherichia coli and Corynebacterium glutamicum are used to overproduce the enzyme L-serine O-acetyltransferase, thereby increasing the yield of this compound .
Chemical Reactions Analysis
Types of Reactions:
Substitution Reactions: Z-O-Acetyl-L-serine undergoes substitution reactions where the acetyl group can be replaced by other functional groups.
Hydrolysis: The acetyl group in this compound can be hydrolyzed under acidic or basic conditions to regenerate L-serine.
Common Reagents and Conditions:
Acetylation: Acetyl-CoA, acetic anhydride, or acetyl chloride in the presence of a base.
Substitution: Thiol-containing compounds such as hydrogen sulfide or thiosulfate.
Hydrolysis: Acidic or basic aqueous solutions.
Major Products:
L-Cysteine: Formed by the substitution of the acetyl group with a thiol group.
L-Serine: Regenerated by the hydrolysis of this compound.
Scientific Research Applications
Chemistry:
- Z-O-Acetyl-L-serine is used as an intermediate in the synthesis of various sulfur-containing compounds, including L-cysteine and its derivatives .
Biology:
- It plays a crucial role in the metabolic pathways of bacteria and plants, particularly in the biosynthesis of cysteine .
Medicine:
- This compound is studied for its potential therapeutic applications in treating diseases related to cysteine metabolism .
Industry:
Comparison with Similar Compounds
L-Serine: The precursor of Z-O-Acetyl-L-serine, involved in various metabolic pathways.
L-Cysteine: The product of this compound metabolism, a sulfur-containing amino acid with numerous biological functions.
O-Acetylhomoserine: Another acetylated amino acid involved in the biosynthesis of methionine.
Uniqueness: this compound is unique due to its specific role in the biosynthesis of L-cysteine. Unlike other acetylated amino acids, it serves as a direct precursor to a sulfur-containing amino acid, making it essential for sulfur metabolism in various organisms .
Properties
IUPAC Name |
(2S)-3-acetyloxy-2-(phenylmethoxycarbonylamino)propanoic acid | |
---|---|---|
Details | Computed by Lexichem TK 2.7.0 (PubChem release 2021.05.07) | |
Source | PubChem | |
URL | https://pubchem.ncbi.nlm.nih.gov | |
Description | Data deposited in or computed by PubChem | |
InChI |
InChI=1S/C13H15NO6/c1-9(15)19-8-11(12(16)17)14-13(18)20-7-10-5-3-2-4-6-10/h2-6,11H,7-8H2,1H3,(H,14,18)(H,16,17)/t11-/m0/s1 | |
Details | Computed by InChI 1.0.6 (PubChem release 2021.05.07) | |
Source | PubChem | |
URL | https://pubchem.ncbi.nlm.nih.gov | |
Description | Data deposited in or computed by PubChem | |
InChI Key |
LILHBTZTKFFRPW-NSHDSACASA-N | |
Details | Computed by InChI 1.0.6 (PubChem release 2021.05.07) | |
Source | PubChem | |
URL | https://pubchem.ncbi.nlm.nih.gov | |
Description | Data deposited in or computed by PubChem | |
Canonical SMILES |
CC(=O)OCC(C(=O)O)NC(=O)OCC1=CC=CC=C1 | |
Details | Computed by OEChem 2.3.0 (PubChem release 2021.05.07) | |
Source | PubChem | |
URL | https://pubchem.ncbi.nlm.nih.gov | |
Description | Data deposited in or computed by PubChem | |
Isomeric SMILES |
CC(=O)OC[C@@H](C(=O)O)NC(=O)OCC1=CC=CC=C1 | |
Details | Computed by OEChem 2.3.0 (PubChem release 2021.05.07) | |
Source | PubChem | |
URL | https://pubchem.ncbi.nlm.nih.gov | |
Description | Data deposited in or computed by PubChem | |
Molecular Formula |
C13H15NO6 | |
Details | Computed by PubChem 2.1 (PubChem release 2021.05.07) | |
Source | PubChem | |
URL | https://pubchem.ncbi.nlm.nih.gov | |
Description | Data deposited in or computed by PubChem | |
Molecular Weight |
281.26 g/mol | |
Details | Computed by PubChem 2.1 (PubChem release 2021.05.07) | |
Source | PubChem | |
URL | https://pubchem.ncbi.nlm.nih.gov | |
Description | Data deposited in or computed by PubChem | |
Retrosynthesis Analysis
AI-Powered Synthesis Planning: Our tool employs the Template_relevance Pistachio, Template_relevance Bkms_metabolic, Template_relevance Pistachio_ringbreaker, Template_relevance Reaxys, Template_relevance Reaxys_biocatalysis model, leveraging a vast database of chemical reactions to predict feasible synthetic routes.
One-Step Synthesis Focus: Specifically designed for one-step synthesis, it provides concise and direct routes for your target compounds, streamlining the synthesis process.
Accurate Predictions: Utilizing the extensive PISTACHIO, BKMS_METABOLIC, PISTACHIO_RINGBREAKER, REAXYS, REAXYS_BIOCATALYSIS database, our tool offers high-accuracy predictions, reflecting the latest in chemical research and data.
Strategy Settings
Precursor scoring | Relevance Heuristic |
---|---|
Min. plausibility | 0.01 |
Model | Template_relevance |
Template Set | Pistachio/Bkms_metabolic/Pistachio_ringbreaker/Reaxys/Reaxys_biocatalysis |
Top-N result to add to graph | 6 |
Feasible Synthetic Routes
Disclaimer and Information on In-Vitro Research Products
Please be aware that all articles and product information presented on BenchChem are intended solely for informational purposes. The products available for purchase on BenchChem are specifically designed for in-vitro studies, which are conducted outside of living organisms. In-vitro studies, derived from the Latin term "in glass," involve experiments performed in controlled laboratory settings using cells or tissues. It is important to note that these products are not categorized as medicines or drugs, and they have not received approval from the FDA for the prevention, treatment, or cure of any medical condition, ailment, or disease. We must emphasize that any form of bodily introduction of these products into humans or animals is strictly prohibited by law. It is essential to adhere to these guidelines to ensure compliance with legal and ethical standards in research and experimentation.