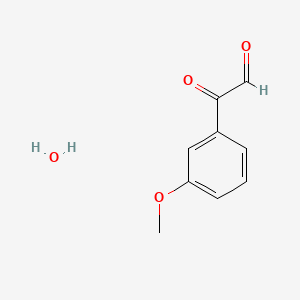
3-Methoxyphenylglyoxal hydrate
Overview
Description
. It is a derivative of phenylglyoxal and contains both aldehyde and ketone functional groups. This compound is typically found in a crystalline hydrate form and is used in various chemical and biological research applications .
Preparation Methods
Synthetic Routes and Reaction Conditions
3-Methoxyphenylglyoxal hydrate can be synthesized through several methods. One common method involves the oxidation of 3-methoxyacetophenone using selenium dioxide as the oxidizing agent . The reaction is typically carried out in an organic solvent such as acetic acid under reflux conditions. The resulting product is then purified through recrystallization.
Another method involves the reaction of 3-methoxybenzaldehyde with glyoxylic acid in the presence of a catalyst. This reaction proceeds under mild conditions and yields this compound as the primary product .
Industrial Production Methods
Industrial production of this compound often involves large-scale oxidation reactions using efficient and cost-effective oxidizing agents. The use of continuous flow reactors and advanced purification techniques ensures high yield and purity of the final product .
Chemical Reactions Analysis
Types of Reactions
3-Methoxyphenylglyoxal hydrate undergoes various chemical reactions, including:
Substitution: The compound can undergo nucleophilic substitution reactions, particularly at the aldehyde group, to form various derivatives.
Common Reagents and Conditions
Oxidizing Agents: Selenium dioxide, potassium permanganate, chromium trioxide.
Reducing Agents: Sodium borohydride, lithium aluminum hydride.
Catalysts: Acidic or basic catalysts depending on the reaction type.
Major Products Formed
Oxidation: Carboxylic acids.
Reduction: Alcohols.
Substitution: Various substituted derivatives.
Scientific Research Applications
3-Methoxyphenylglyoxal hydrate is widely used in scientific research due to its versatile chemical properties. Some of its applications include:
Mechanism of Action
The mechanism of action of 3-methoxyphenylglyoxal hydrate involves its interaction with nucleophilic sites in biological molecules. The compound can form covalent bonds with amino acids such as arginine, leading to the modification of protein structure and function . This property makes it useful in studying enzyme mechanisms and protein interactions.
Comparison with Similar Compounds
Similar Compounds
Phenylglyoxal: Similar structure but lacks the methoxy group.
Methylglyoxal: Contains a methyl group instead of a phenyl group.
3,4-Dihydroxyphenylacetaldehyde: Contains hydroxyl groups instead of a methoxy group.
Uniqueness
3-Methoxyphenylglyoxal hydrate is unique due to the presence of the methoxy group, which imparts distinct chemical properties and reactivity compared to its analogs. This makes it particularly useful in specific synthetic and research applications where the methoxy group plays a crucial role .
Properties
IUPAC Name |
2-(3-methoxyphenyl)-2-oxoacetaldehyde;hydrate | |
---|---|---|
Source | PubChem | |
URL | https://pubchem.ncbi.nlm.nih.gov | |
Description | Data deposited in or computed by PubChem | |
InChI |
InChI=1S/C9H8O3.H2O/c1-12-8-4-2-3-7(5-8)9(11)6-10;/h2-6H,1H3;1H2 | |
Source | PubChem | |
URL | https://pubchem.ncbi.nlm.nih.gov | |
Description | Data deposited in or computed by PubChem | |
InChI Key |
LDMLATLMTDGBHP-UHFFFAOYSA-N | |
Source | PubChem | |
URL | https://pubchem.ncbi.nlm.nih.gov | |
Description | Data deposited in or computed by PubChem | |
Canonical SMILES |
COC1=CC=CC(=C1)C(=O)C=O.O | |
Source | PubChem | |
URL | https://pubchem.ncbi.nlm.nih.gov | |
Description | Data deposited in or computed by PubChem | |
Molecular Formula |
C9H10O4 | |
Source | PubChem | |
URL | https://pubchem.ncbi.nlm.nih.gov | |
Description | Data deposited in or computed by PubChem | |
DSSTOX Substance ID |
DTXSID40611093 | |
Record name | (3-Methoxyphenyl)(oxo)acetaldehyde--water (1/1) | |
Source | EPA DSSTox | |
URL | https://comptox.epa.gov/dashboard/DTXSID40611093 | |
Description | DSSTox provides a high quality public chemistry resource for supporting improved predictive toxicology. | |
Molecular Weight |
182.17 g/mol | |
Source | PubChem | |
URL | https://pubchem.ncbi.nlm.nih.gov | |
Description | Data deposited in or computed by PubChem | |
CAS No. |
118888-62-3 | |
Record name | (3-Methoxyphenyl)(oxo)acetaldehyde--water (1/1) | |
Source | EPA DSSTox | |
URL | https://comptox.epa.gov/dashboard/DTXSID40611093 | |
Description | DSSTox provides a high quality public chemistry resource for supporting improved predictive toxicology. | |
Retrosynthesis Analysis
AI-Powered Synthesis Planning: Our tool employs the Template_relevance Pistachio, Template_relevance Bkms_metabolic, Template_relevance Pistachio_ringbreaker, Template_relevance Reaxys, Template_relevance Reaxys_biocatalysis model, leveraging a vast database of chemical reactions to predict feasible synthetic routes.
One-Step Synthesis Focus: Specifically designed for one-step synthesis, it provides concise and direct routes for your target compounds, streamlining the synthesis process.
Accurate Predictions: Utilizing the extensive PISTACHIO, BKMS_METABOLIC, PISTACHIO_RINGBREAKER, REAXYS, REAXYS_BIOCATALYSIS database, our tool offers high-accuracy predictions, reflecting the latest in chemical research and data.
Strategy Settings
Precursor scoring | Relevance Heuristic |
---|---|
Min. plausibility | 0.01 |
Model | Template_relevance |
Template Set | Pistachio/Bkms_metabolic/Pistachio_ringbreaker/Reaxys/Reaxys_biocatalysis |
Top-N result to add to graph | 6 |
Feasible Synthetic Routes
Disclaimer and Information on In-Vitro Research Products
Please be aware that all articles and product information presented on BenchChem are intended solely for informational purposes. The products available for purchase on BenchChem are specifically designed for in-vitro studies, which are conducted outside of living organisms. In-vitro studies, derived from the Latin term "in glass," involve experiments performed in controlled laboratory settings using cells or tissues. It is important to note that these products are not categorized as medicines or drugs, and they have not received approval from the FDA for the prevention, treatment, or cure of any medical condition, ailment, or disease. We must emphasize that any form of bodily introduction of these products into humans or animals is strictly prohibited by law. It is essential to adhere to these guidelines to ensure compliance with legal and ethical standards in research and experimentation.