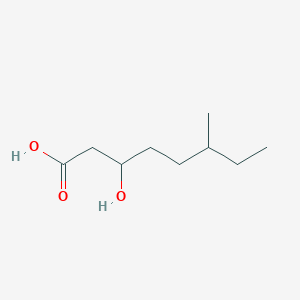
3-Hydroxy-6-methyloctanoic acid
Overview
Description
3-Hydroxy-6-methyloctanoic acid is an organic compound with the molecular formula C9H18O3 and a molecular weight of 174.24 g/mol . It is a colorless to slightly yellow liquid with a distinctive odor. This compound is slightly soluble in water but soluble in organic solvents such as ethanol and chloroform .
Preparation Methods
Synthetic Routes and Reaction Conditions
3-Hydroxy-6-methyloctanoic acid can be synthesized through various methods. One common synthetic route involves the reaction of octanal with hydrogen cyanide to form octanenitrile, which is then hydrolyzed to produce the desired hydroxy acid . The reaction conditions typically involve:
Reactants: Octanal and hydrogen cyanide
Catalysts: Acid or base catalysts for hydrolysis
Solvents: Organic solvents like ethanol or chloroform
Temperature: Controlled temperatures to optimize yield and purity
Industrial Production Methods
Industrial production of this compound often involves similar synthetic routes but on a larger scale. The process may include:
Batch or continuous reactors: To handle large volumes of reactants
Purification steps: Such as distillation or crystallization to ensure high purity
Safety measures: To handle hazardous chemicals like hydrogen cyanide
Chemical Reactions Analysis
Types of Reactions
3-Hydroxy-6-methyloctanoic acid undergoes various chemical reactions, including:
Oxidation: The hydroxyl group can be oxidized to form a ketone or carboxylic acid.
Reduction: The carboxylic acid group can be reduced to form an alcohol.
Substitution: The hydroxyl group can be substituted with other functional groups.
Common Reagents and Conditions
Oxidizing agents: Such as potassium permanganate or chromium trioxide
Reducing agents: Such as lithium aluminum hydride or sodium borohydride
Solvents: Organic solvents like dichloromethane or tetrahydrofuran
Temperature and pH: Controlled to optimize reaction rates and yields
Major Products
Oxidation: Formation of 3-keto-6-methyloctanoic acid or 3-carboxy-6-methyloctanoic acid
Reduction: Formation of 3-hydroxy-6-methyloctanol
Substitution: Formation of various substituted derivatives depending on the reagents used
Scientific Research Applications
3-Hydroxy-6-methyloctanoic acid has diverse applications in scientific research, including:
Chemistry: Used as an intermediate in the synthesis of complex organic molecules.
Biology: Studied for its role in metabolic pathways and as a potential biomarker.
Medicine: Investigated for its potential therapeutic properties and as a building block for drug synthesis.
Industry: Utilized in the production of biofuels, surfactants, and other industrial chemicals.
Mechanism of Action
The mechanism of action of 3-Hydroxy-6-methyloctanoic acid involves its interaction with specific molecular targets and pathways. For example, it can act as a precursor in the biosynthesis of cyclic lipopeptide antibiotics, where it is incorporated into the lipid moiety of the molecule . This incorporation can affect the antibiotic’s ability to disrupt bacterial cell membranes, leading to bacterial cell death .
Comparison with Similar Compounds
Similar Compounds
- 3-Hydroxy-6-methylheptanoic acid
- 3-Hydroxy-6-methylnonanoic acid
- 3-Hydroxy-6-methylundecanoic acid
Uniqueness
3-Hydroxy-6-methyloctanoic acid is unique due to its specific chain length and functional groups, which confer distinct chemical and physical properties. Compared to similar compounds, it may exhibit different reactivity and biological activity, making it valuable for specific applications in research and industry .
Properties
IUPAC Name |
3-hydroxy-6-methyloctanoic acid | |
---|---|---|
Details | Computed by LexiChem 2.6.6 (PubChem release 2019.06.18) | |
Source | PubChem | |
URL | https://pubchem.ncbi.nlm.nih.gov | |
Description | Data deposited in or computed by PubChem | |
InChI |
InChI=1S/C9H18O3/c1-3-7(2)4-5-8(10)6-9(11)12/h7-8,10H,3-6H2,1-2H3,(H,11,12) | |
Details | Computed by InChI 1.0.5 (PubChem release 2019.06.18) | |
Source | PubChem | |
URL | https://pubchem.ncbi.nlm.nih.gov | |
Description | Data deposited in or computed by PubChem | |
InChI Key |
CKOHYUUVZVLYIP-UHFFFAOYSA-N | |
Details | Computed by InChI 1.0.5 (PubChem release 2019.06.18) | |
Source | PubChem | |
URL | https://pubchem.ncbi.nlm.nih.gov | |
Description | Data deposited in or computed by PubChem | |
Canonical SMILES |
CCC(C)CCC(CC(=O)O)O | |
Details | Computed by OEChem 2.1.5 (PubChem release 2019.06.18) | |
Source | PubChem | |
URL | https://pubchem.ncbi.nlm.nih.gov | |
Description | Data deposited in or computed by PubChem | |
Molecular Formula |
C9H18O3 | |
Details | Computed by PubChem 2.1 (PubChem release 2019.06.18) | |
Source | PubChem | |
URL | https://pubchem.ncbi.nlm.nih.gov | |
Description | Data deposited in or computed by PubChem | |
Molecular Weight |
174.24 g/mol | |
Details | Computed by PubChem 2.1 (PubChem release 2021.05.07) | |
Source | PubChem | |
URL | https://pubchem.ncbi.nlm.nih.gov | |
Description | Data deposited in or computed by PubChem | |
Retrosynthesis Analysis
AI-Powered Synthesis Planning: Our tool employs the Template_relevance Pistachio, Template_relevance Bkms_metabolic, Template_relevance Pistachio_ringbreaker, Template_relevance Reaxys, Template_relevance Reaxys_biocatalysis model, leveraging a vast database of chemical reactions to predict feasible synthetic routes.
One-Step Synthesis Focus: Specifically designed for one-step synthesis, it provides concise and direct routes for your target compounds, streamlining the synthesis process.
Accurate Predictions: Utilizing the extensive PISTACHIO, BKMS_METABOLIC, PISTACHIO_RINGBREAKER, REAXYS, REAXYS_BIOCATALYSIS database, our tool offers high-accuracy predictions, reflecting the latest in chemical research and data.
Strategy Settings
Precursor scoring | Relevance Heuristic |
---|---|
Min. plausibility | 0.01 |
Model | Template_relevance |
Template Set | Pistachio/Bkms_metabolic/Pistachio_ringbreaker/Reaxys/Reaxys_biocatalysis |
Top-N result to add to graph | 6 |
Feasible Synthetic Routes
Disclaimer and Information on In-Vitro Research Products
Please be aware that all articles and product information presented on BenchChem are intended solely for informational purposes. The products available for purchase on BenchChem are specifically designed for in-vitro studies, which are conducted outside of living organisms. In-vitro studies, derived from the Latin term "in glass," involve experiments performed in controlled laboratory settings using cells or tissues. It is important to note that these products are not categorized as medicines or drugs, and they have not received approval from the FDA for the prevention, treatment, or cure of any medical condition, ailment, or disease. We must emphasize that any form of bodily introduction of these products into humans or animals is strictly prohibited by law. It is essential to adhere to these guidelines to ensure compliance with legal and ethical standards in research and experimentation.