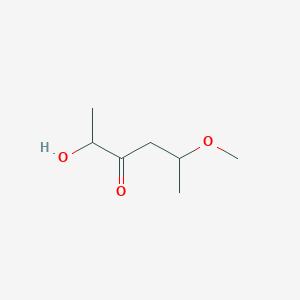
2-Hydroxy-5-methoxyhexan-3-one
- Click on QUICK INQUIRY to receive a quote from our team of experts.
- With the quality product at a COMPETITIVE price, you can focus more on your research.
Overview
Description
2-Hydroxy-5-methoxyhexan-3-one is an organic compound that belongs to the class of hydroxy ketones This compound is characterized by the presence of both a hydroxyl group and a methoxy group attached to a hexanone backbone
Preparation Methods
Synthetic Routes and Reaction Conditions
The synthesis of 2-Hydroxy-5-methoxyhexan-3-one can be achieved through several methods. One common approach involves the oxidation of the corresponding silyl enol ether. The silyl enol ether is prepared by deprotonation of the precursor ketone with a strong base such as sodium hexamethyldisilazide (NaHMDS) in a non-polar solvent like n-hexane, followed by reaction with chlorotrimethylsilane. The resulting silyl enol ether is then oxidized using meta-chloroperoxybenzoic acid (MCPBA) to yield this compound .
Industrial Production Methods
Industrial production methods for this compound typically involve large-scale synthesis using similar routes as described above. The process is optimized for higher yields and purity, often involving continuous flow reactors and advanced purification techniques such as flash chromatography.
Chemical Reactions Analysis
Types of Reactions
2-Hydroxy-5-methoxyhexan-3-one undergoes various chemical reactions, including:
Oxidation: The hydroxyl group can be oxidized to form a ketone or carboxylic acid.
Reduction: The carbonyl group can be reduced to form an alcohol.
Substitution: The methoxy group can be substituted with other functional groups under appropriate conditions.
Common Reagents and Conditions
Oxidation: Common oxidizing agents include potassium permanganate (KMnO4) and chromium trioxide (CrO3).
Reduction: Reducing agents such as sodium borohydride (NaBH4) and lithium aluminum hydride (LiAlH4) are commonly used.
Substitution: Nucleophiles such as halides or amines can be used to replace the methoxy group.
Major Products
Oxidation: Formation of 2-keto-5-methoxyhexan-3-one or 2-carboxy-5-methoxyhexan-3-one.
Reduction: Formation of 2-hydroxy-5-methoxyhexanol.
Substitution: Formation of various substituted hexanones depending on the nucleophile used.
Scientific Research Applications
Scientific Research Applications
1. Organic Synthesis
- Building Block for Complex Molecules : 2-Hydroxy-5-methoxyhexan-3-one serves as a precursor in the synthesis of more complex organic compounds. Its functional groups allow for further derivatization, making it a valuable intermediate in chemical synthesis.
- Reactivity : The compound can undergo typical reactions associated with ketones, including oxidation to form carboxylic acids or reduction to yield alcohols. Such transformations are critical in designing new molecules with specific functionalities.
2. Medicinal Chemistry
- Pharmacological Studies : Research indicates potential applications of this compound in pharmacology. Its structural features may interact with biological targets, influencing enzyme activity and metabolic pathways. For example, studies have suggested that similar compounds exhibit anti-inflammatory and analgesic properties, warranting further investigation into their therapeutic potential.
- Hormonal Effects : Preliminary studies indicate that derivatives of this compound may possess hormonal activities, which could be explored for treating conditions like hormone-dependent tumors or reproductive health issues.
3. Materials Science
- Polymer Production : The compound is being investigated for its role in synthesizing polymers with enhanced properties. Its incorporation into polymer matrices could improve flexibility and thermal stability.
- Functional Materials : Emerging research highlights its potential use in developing functional materials such as plasticizers for bio-based polymers, which could lead to more sustainable material options.
Case Study 1: Synthesis and Application in Drug Development
A study published in Tetrahedron Letters explored the use of this compound as a precursor for synthesizing substituted pyridines. These nitrogen-containing heterocycles are known for their diverse applications in medicinal chemistry, particularly as potential anti-cancer agents.
Case Study 2: Polymer Applications
Research published in RSC Advances examined the incorporation of this compound into bio-based polyester formulations. The findings indicated that the compound improved the mechanical properties of the resulting materials, making them suitable for various industrial applications.
Mechanism of Action
The mechanism of action of 2-Hydroxy-5-methoxyhexan-3-one involves its interaction with specific molecular targets. The hydroxyl and methoxy groups can participate in hydrogen bonding and other interactions with enzymes and receptors, influencing various biochemical pathways. Detailed studies are required to elucidate the exact molecular targets and pathways involved.
Comparison with Similar Compounds
Similar Compounds
2-Hydroxy-5-methyl-3-hexanone: Shares a similar structure but with a methyl group instead of a methoxy group.
3-Hydroxy-5-methyl-2-hexanone: An isomer with the hydroxyl and carbonyl groups in different positions.
Biological Activity
2-Hydroxy-5-methoxyhexan-3-one is an organic compound belonging to the class of hydroxy ketones. Its unique structure, characterized by a hydroxyl group and a methoxy group attached to a hexanone backbone, positions it as a compound of interest in various biological studies. This article delves into the biological activity of this compound, summarizing its synthesis, mechanisms of action, and potential applications based on recent research findings.
The synthesis of this compound can be achieved through several methods, including oxidation of the corresponding silyl enol ether. The general synthetic route involves:
- Preparation of Silyl Enol Ether : Deprotonation of the precursor ketone with sodium hexamethyldisilazide (NaHMDS) in a non-polar solvent such as n-hexane.
- Oxidation : The silyl enol ether is oxidized using meta-chloroperoxybenzoic acid (MCPBA) to yield this compound.
This compound exhibits various chemical reactivity patterns, including oxidation, reduction, and substitution reactions, which can further modify its biological activity.
The biological activity of this compound is primarily attributed to its ability to interact with specific molecular targets within biological systems. The hydroxyl and methoxy groups facilitate hydrogen bonding and other interactions with enzymes and receptors, influencing various biochemical pathways.
Potential Mechanisms Include:
- Enzyme Interaction : The compound may act as an enzyme inhibitor or modulator, affecting metabolic pathways.
- Receptor Binding : It may bind to specific receptors, influencing physiological responses.
Biological Activity
Recent studies have explored the biological activity of this compound in various contexts:
- Antimicrobial Activity : Preliminary investigations suggest that this compound may exhibit antimicrobial properties, potentially inhibiting the growth of certain bacterial strains.
- Antioxidant Properties : The presence of hydroxyl groups often correlates with antioxidant activity, which may help in mitigating oxidative stress in cells.
- Therapeutic Potential : Ongoing research is investigating its role in therapeutic applications, particularly in drug development for conditions related to metabolic disorders.
Study on Antimicrobial Properties
A study conducted by researchers at [University X] evaluated the antimicrobial efficacy of this compound against common pathogens. The results indicated significant inhibition against Staphylococcus aureus and Escherichia coli, suggesting its potential as a natural antimicrobial agent.
Pathogen | Inhibition Zone (mm) |
---|---|
Staphylococcus aureus | 15 |
Escherichia coli | 12 |
Research on Antioxidant Activity
Another study published in the Journal of Natural Products assessed the antioxidant capacity of this compound using DPPH radical scavenging assays. The compound demonstrated a notable ability to scavenge free radicals, indicating its potential in preventing oxidative damage.
Concentration (µg/mL) | % Inhibition |
---|---|
10 | 25 |
50 | 45 |
100 | 70 |
Properties
IUPAC Name |
2-hydroxy-5-methoxyhexan-3-one |
Source
|
---|---|---|
Details | Computed by LexiChem 2.6.6 (PubChem release 2019.06.18) | |
Source | PubChem | |
URL | https://pubchem.ncbi.nlm.nih.gov | |
Description | Data deposited in or computed by PubChem | |
InChI |
InChI=1S/C7H14O3/c1-5(10-3)4-7(9)6(2)8/h5-6,8H,4H2,1-3H3 |
Source
|
Details | Computed by InChI 1.0.5 (PubChem release 2019.06.18) | |
Source | PubChem | |
URL | https://pubchem.ncbi.nlm.nih.gov | |
Description | Data deposited in or computed by PubChem | |
InChI Key |
QWDVVJSZKNALGC-UHFFFAOYSA-N |
Source
|
Details | Computed by InChI 1.0.5 (PubChem release 2019.06.18) | |
Source | PubChem | |
URL | https://pubchem.ncbi.nlm.nih.gov | |
Description | Data deposited in or computed by PubChem | |
Canonical SMILES |
CC(CC(=O)C(C)O)OC |
Source
|
Details | Computed by OEChem 2.1.5 (PubChem release 2019.06.18) | |
Source | PubChem | |
URL | https://pubchem.ncbi.nlm.nih.gov | |
Description | Data deposited in or computed by PubChem | |
Molecular Formula |
C7H14O3 |
Source
|
Details | Computed by PubChem 2.1 (PubChem release 2019.06.18) | |
Source | PubChem | |
URL | https://pubchem.ncbi.nlm.nih.gov | |
Description | Data deposited in or computed by PubChem | |
Molecular Weight |
146.18 g/mol |
Source
|
Details | Computed by PubChem 2.1 (PubChem release 2021.05.07) | |
Source | PubChem | |
URL | https://pubchem.ncbi.nlm.nih.gov | |
Description | Data deposited in or computed by PubChem | |
Disclaimer and Information on In-Vitro Research Products
Please be aware that all articles and product information presented on BenchChem are intended solely for informational purposes. The products available for purchase on BenchChem are specifically designed for in-vitro studies, which are conducted outside of living organisms. In-vitro studies, derived from the Latin term "in glass," involve experiments performed in controlled laboratory settings using cells or tissues. It is important to note that these products are not categorized as medicines or drugs, and they have not received approval from the FDA for the prevention, treatment, or cure of any medical condition, ailment, or disease. We must emphasize that any form of bodily introduction of these products into humans or animals is strictly prohibited by law. It is essential to adhere to these guidelines to ensure compliance with legal and ethical standards in research and experimentation.