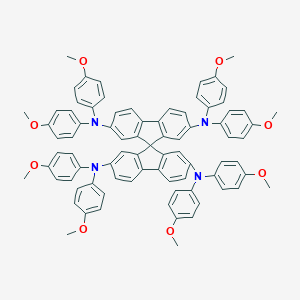
Spiro-OMeTAD
Overview
Description
Spiro-MeOTAD is a stable and efficient hole-transport material in organic light-emitting devices and in solid-state dye-sensitized solar cells (ssDSSCs). It yields higher ssDSSC efficiency compared to the liquid electrolyte for DSSC solar cells due to its reasonable charge carrier mobility and its amorphous nature and high solubility, which enables excellent infiltration into mesoporous titania films. Neutral spiro-MeOTAD absorbs light in the UV region of the spectrum, while its oxidized forms exhibit strong absorptions throughout the visible and near-infrared ranges.
Mechanism of Action
Target of Action
The primary target of Spiro-OMeTAD is to serve as a hole transport layer (HTL) in perovskite solar cells . The choice of HTLs is a key factor for achieving efficient and stable PSCs . This compound has been proven to be the most suitable small molecule HTL material in n–i–p PSCs .
Mode of Action
This compound interacts with its targets primarily through oxidation . The oxidation of this compound is facilitated by complexation between the zinc cation and tert-butylpyridine (tBP) even in the absence of oxygen and light . In addition, electron transfer from neutral this compound to radicals provides free holes to instantly increase the conductivity and work function .
Biochemical Pathways
The key biochemical pathway affected by this compound involves the oxidation process . The oxygen oxidizes the this compound film while LiTFSI acts as a mediator in the reaction between O2 and this compound, producing a TFSI-stabilized radical cation along with lithium oxides .
Pharmacokinetics
While the term “pharmacokinetics” is typically used in the context of drug metabolism, in the case of this compound, we can discuss its properties related to its distribution and action within the PSC. Single crystals of Spiro(TFSI)2 were grown, and the optical and electronic properties were characterized and compared with neutral this compound .
Result of Action
The result of this compound’s action is an increase in the conductivity and hole mobility of the HTL in PSCs . This leads to an improvement in the power conversion efficiency (PCE) of PSCs .
Action Environment
The action of this compound is significantly influenced by environmental factors, including oxygen and moisture levels . The traditional oxidation process relies on the slow ingress of O2 into and diffusion through this compound:LiTFSI, which would usually take several hours or days and is heavily dependent on ambient conditions .
Biological Activity
Spiro-OMeTAD (2,2',7,7'-tetrakis-(N,N-di-p-methoxyphenylamine)-9,9'-spirobifluorene) is a small organic molecule widely recognized as a hole transport material (HTM) in perovskite solar cells (PSCs). Its unique structure and electronic properties have made it a focal point of research aimed at improving the efficiency and stability of PSCs. This article delves into the biological activity of this compound, exploring its interactions, modifications, and applications in various fields.
Molecular Structure
This compound features a spirobifluorene core that facilitates efficient charge transport due to its robust π-conjugated system. The presence of methoxy groups enhances solubility and electronic properties, making it an ideal candidate for HTMs.
Key Properties
- Molecular Weight : 404.5 g/mol
- Energy Levels :
- Highest Occupied Molecular Orbital (HOMO): ~5.14 eV
- Lowest Unoccupied Molecular Orbital (LUMO): ~3.85 eV
- Solubility : Soluble in common organic solvents like chlorobenzene.
This compound exhibits notable biological activity primarily through its role as an electron donor in various chemical reactions. Its ability to donate electrons makes it effective in photochemical applications, including solar energy conversion.
Case Studies
- Doping Effects : Research indicates that doping this compound with materials such as lithium bis(trifluoromethanesulfonyl)imide (Li-TFSI) enhances its conductivity and stability in PSCs. The introduction of dopants modifies the energy levels, improving hole transport efficiency .
- Radical Formation : Studies using electron spin resonance (ESR) spectroscopy have shown that doping this compound with triphenylphosphine (TPP) leads to the formation of radical species, which are critical for enhancing the oxidation process of this compound. This transformation is essential for optimizing its performance in solar cells .
Biological Interactions
While primarily studied for its electronic properties, this compound has shown potential interactions with biological systems:
- DNA Binding : Recent studies indicate that dendrimers incorporating this compound can effectively bind and compact DNA, suggesting potential applications in gene delivery systems .
- Cellular Toxicity : Investigations into the cytotoxicity of this compound have revealed minimal adverse effects on mammalian cells at low concentrations, indicating safety for potential biomedical applications .
Doping Strategies
The effectiveness of this compound as an HTM can be significantly enhanced through various doping strategies:
- Common Dopants : Li-TFSI and TPP are among the most studied dopants that improve charge transport properties.
- Emerging Dopants : New materials such as 2,3,5,6-tetrafluoro-7,7,8,8-tetracyanoquinodimethane (F4TCNQ) have been explored to enhance stability without compromising efficiency .
Stability Improvements
Research indicates that modifications to the molecular structure or the incorporation of alternative dopants can lead to improved thermal and photostability of this compound-based devices. For instance, studies show that devices utilizing modified this compound retain up to 80% efficiency after prolonged exposure to operational conditions .
Data Summary
Property | Value |
---|---|
Molecular Weight | 404.5 g/mol |
HOMO | ~5.14 eV |
LUMO | ~3.85 eV |
Conductivity (doped) | Up to 2.79 × 10⁻³ S/cm |
Stability Retention | Up to 80% after 1000 hours |
Scientific Research Applications
Role in Perovskite Solar Cells
Hole Transport Layer (HTL):
Spiro-OMeTAD serves as a crucial HTL in PSCs, facilitating the extraction of holes from the perovskite layer to the anode. The efficiency of PSCs has significantly improved with the incorporation of this compound due to its high hole mobility and favorable energy levels.
Recent Advances
- Lithium-Free Doping Strategy: Researchers have developed a lithium-free doping method using Eu(TFSI) salts that enhances the conductivity and stability of this compound without the need for time-consuming air oxidation. This method has led to PSCs achieving power conversion efficiencies (PCE) of up to 25.45%, surpassing traditional lithium-doped configurations which typically yield lower efficiencies .
- Stability Improvements: Devices utilizing O2-derived this compound HTLs demonstrated a significant retention of 90% of their initial efficiency after 1000 hours of operation, indicating enhanced long-term stability compared to conventional methods .
Comparison with Other Hole Conductors
This compound has been compared with other HTMs such as P3HT and PTAA in solid-state dye-sensitized solar cells. Studies indicate that while this compound offers superior performance in terms of efficiency and stability, it also presents challenges related to its synthesis and processing compared to P3HT .
Property | This compound | P3HT |
---|---|---|
Hole Mobility | High | Moderate |
Thermal Stability | Good | Moderate |
Efficiency in PSCs | High (>25%) | Lower (~20%) |
Doping Requirements | Often requires LiTFSI | Less stringent |
Biochemical Applications
Beyond photovoltaics, this compound is being explored for its potential in biochemical applications. Its redox properties allow for modifications that can be utilized in biosensors and bioelectronic devices, where electron transfer capabilities are critical. This adaptability stems from its deep redox potential, making it suitable for applications involving enzyme interactions and electrochemical sensing .
Future Directions and Challenges
While this compound has proven effective in enhancing the performance of PSCs, there are ongoing challenges:
- Synthesis Complexity: The production of high-purity this compound remains complex and costly.
- Environmental Stability: Although recent advancements have improved its stability, further research is needed to address degradation mechanisms under operational conditions.
Case Studies
Case Study 1: Enhanced Efficiency through Novel Doping
A study conducted by researchers at Northwestern Polytechnical University demonstrated that by employing a novel doping strategy using Eu(TFSI), they achieved a PCE of 25.45% in this compound-based PSCs. This study highlighted the importance of optimizing HTL properties for commercial viability .
Case Study 2: Comparative Analysis with PTAA
A comprehensive review comparing this compound with PTAA revealed significant differences in electronic properties and degradation pathways. This analysis underscored the potential for this compound to outperform PTAA under specific conditions, particularly regarding long-term stability and efficiency in large-area devices .
Chemical Reactions Analysis
Oxidation Reactions
Spiro-OMeTAD requires oxidation to achieve p-type doping, which enhances conductivity and aligns its work function with perovskite layers.
(a) Superoxide Radical-Mediated Oxidation
- Mechanism : Superoxide radicals (- O₂⁻) generated via europium(III) bis(trifluoromethanesulfonyl)imide [Eu(TFSI)₂] catalyze the oxidation of this compound in solution.
- Evidence :
- Impact : Achieves conductivity of 0.1–0.3 mS/cm and PSC efficiency >25% without post-treatment .
(b) Hot Pure Oxygen Treatment (HPOT)
- Conditions : Heating this compound films in pure O₂ at 70°C for 10 minutes .
- Outcome :
- Accelerates oxidation kinetics by 10× vs. ambient air.
- Reduces LiTFSI aggregation, enhancing film homogeneity.
(c) Traditional LiTFSI/Air Exposure
Doping Mechanisms
Dopants modulate this compound’s electronic structure via redox reactions.
(a) Lithium Salts (LiTFSI)
(b) Zinc Salts (Zn(TFSI)₂)
- Mechanism : Zn²⁺ facilitates direct electron transfer without O₂, forming this compound⁺ .
- Advantage : Reduces reliance on ambient conditions, improving reproducibility.
(c) Manganese Complex Doping
- Process : Mn³⁺/Mn²⁺ redox pair oxidizes this compound, confirmed by UV-vis spectra .
- Result : 17.62% PCE in PSCs vs. 15.3% for undoped HTMs .
Electrochemical Reactions
In aqueous environments, this compound undergoes reversible redox reactions:
Implications : Critical for stability in moisture-rich conditions .
Redox Reversibility and Degradation
- Reduction by Iodine : Oxidized this compound⁺ reverts to neutral form in the presence of I⁻, forming I₂ .
- Degradation Pathways :
Comparative Analysis of Oxidation Methods
Synthetic Modifications
Q & A
Basic Research Questions
Q. What is the role of Spiro-OMeTAD in perovskite solar cells (PSCs), and how does its molecular structure enable this function?
this compound serves as a hole-transporting material (HTL) in PSCs, facilitating efficient charge extraction from the perovskite layer to the electrode. Its spirobifluorene core and triarylamine moieties provide a stable radical cation state, ensuring high hole mobility and energy level alignment with perovskite absorbers like CH3NH3PbI3 . The methoxy (-OMe) substituents further enhance solubility and film-forming properties, critical for uniform layer deposition .
Q. What experimental methods are used to characterize the conductivity and oxidation state of this compound?
Researchers employ cyclic voltammetry to determine oxidation potentials and UV-vis spectroscopy to monitor charge-transfer transitions. Electron paramagnetic resonance (EPR) spectroscopy is critical for detecting radical cations (giso ≈ 2.0033), confirming doping efficiency under anaerobic or illuminated conditions . Additionally, conductivity measurements via four-probe techniques and photoelectron spectroscopy (UPS/XPS) assess work function tunability .
Q. How do researchers optimize this compound thickness in device architectures?
Layer thickness is optimized using simulation tools like SCAPS-1D, which models charge transport and recombination. Experimental validation involves spin-coating this compound at varying speeds (e.g., 2000–6000 rpm) to achieve 100–300 nm films. A balance is sought: thinner layers reduce series resistance but may cause pinholes, while thicker layers impede charge extraction .
Advanced Research Questions
Q. What are the limitations of conventional this compound doping strategies, and how can they be addressed?
Traditional doping with hygroscopic Li-TFSI and volatile 4-tert-butylpyridine (tBP) causes instability due to moisture ingress and dopant migration. Advanced approaches include:
- Ion-modulated radical doping : Stable organic radicals (e.g., Spiro-TTB) combined with ionic salts (e.g., FK209) decouple conductivity and work function tuning, achieving >25% PCE and enhanced stability .
- Additive engineering : Incorporating hydrophobic CNT:TiO2 or star-shaped polymers passivate defects and block moisture, retaining 72% PCE after 1000 hours .
Q. How does substituent positioning in this compound derivatives influence photovoltaic performance?
Methoxy group placement alters molecular packing and charge transport. For example, ortho-OMe substituents (vs. para) improve intermolecular interactions, boosting Jsc (21.2 mA/cm²) and PCE (16.7%) by reducing recombination and enhancing hole mobility . Computational modeling (DFT) and grazing-incidence X-ray diffraction (GI-XRD) are used to correlate substituent effects with crystallinity and film morphology .
Q. What methodologies resolve contradictions in reported doping kinetics and this compound oxidation mechanisms?
Discrepancies arise from ambient vs. inert conditions. Controlled EPR studies under anaerobic environments reveal that oxidation occurs even without O2, driven by light-induced charge separation. Kinetics vary with dopant type: Cu(TFSI)2 accelerates radical formation compared to Li-TFSI, necessitating in situ monitoring during device fabrication .
Q. How can simulation tools like SCAPS-1D guide the selection of compatible transport layers for this compound-based devices?
SCAPS-1D models band alignment, carrier concentration, and interface defects. For example, pairing this compound with SnO2 ETLs in CsSnI3 PSCs maximizes efficiency (20.2%) by optimizing electron/hole extraction barriers. Experimental validation involves J-V curve analysis and impedance spectroscopy to verify simulated outcomes .
Q. What strategies mitigate interfacial recombination at the this compound/perovskite interface?
- Surface passivation : Treating perovskite with PbI2 or 2D/3D heterostructures reduces trap states.
- Interfacial layers : Inserting ultrathin MoO3 or PTAA between this compound and Au improves energy-level cascading, increasing Voc by ~0.1 V .
- Dopant gradients : Graded doping profiles (higher dopant concentration near electrodes) enhance built-in potential and reduce shunt losses .
Q. Methodological Guidance
Q. How should researchers design experiments to evaluate this compound stability under operational conditions?
- Accelerated aging tests : Expose devices to 85°C/85% RH or continuous 1-sun illumination (ISOS protocols).
- In situ characterization: Use Raman spectroscopy or XRD to monitor structural degradation.
- Encapsulation benchmarking : Compare PCE retention with/without atomic-layer-deposited Al2O3 barriers .
Q. What statistical approaches are recommended for analyzing performance variations in this compound-based devices?
Properties
IUPAC Name |
2-N,2-N,2-N',2-N',7-N,7-N,7-N',7-N'-octakis(4-methoxyphenyl)-9,9'-spirobi[fluorene]-2,2',7,7'-tetramine | |
---|---|---|
Source | PubChem | |
URL | https://pubchem.ncbi.nlm.nih.gov | |
Description | Data deposited in or computed by PubChem | |
InChI |
InChI=1S/C81H68N4O8/c1-86-65-29-9-53(10-30-65)82(54-11-31-66(87-2)32-12-54)61-25-45-73-74-46-26-62(83(55-13-33-67(88-3)34-14-55)56-15-35-68(89-4)36-16-56)50-78(74)81(77(73)49-61)79-51-63(84(57-17-37-69(90-5)38-18-57)58-19-39-70(91-6)40-20-58)27-47-75(79)76-48-28-64(52-80(76)81)85(59-21-41-71(92-7)42-22-59)60-23-43-72(93-8)44-24-60/h9-52H,1-8H3 | |
Source | PubChem | |
URL | https://pubchem.ncbi.nlm.nih.gov | |
Description | Data deposited in or computed by PubChem | |
InChI Key |
XDXWNHPWWKGTKO-UHFFFAOYSA-N | |
Source | PubChem | |
URL | https://pubchem.ncbi.nlm.nih.gov | |
Description | Data deposited in or computed by PubChem | |
Canonical SMILES |
COC1=CC=C(C=C1)N(C2=CC=C(C=C2)OC)C3=CC4=C(C=C3)C5=C(C46C7=C(C=CC(=C7)N(C8=CC=C(C=C8)OC)C9=CC=C(C=C9)OC)C1=C6C=C(C=C1)N(C1=CC=C(C=C1)OC)C1=CC=C(C=C1)OC)C=C(C=C5)N(C1=CC=C(C=C1)OC)C1=CC=C(C=C1)OC | |
Source | PubChem | |
URL | https://pubchem.ncbi.nlm.nih.gov | |
Description | Data deposited in or computed by PubChem | |
Molecular Formula |
C81H68N4O8 | |
Source | PubChem | |
URL | https://pubchem.ncbi.nlm.nih.gov | |
Description | Data deposited in or computed by PubChem | |
DSSTOX Substance ID |
DTXSID90583231 | |
Record name | N~2~,N~2~,N~2'~,N~2'~,N~7~,N~7~,N~7'~,N~7'~-Octakis(4-methoxyphenyl)-9,9'-spirobi[fluorene]-2,2',7,7'-tetramine | |
Source | EPA DSSTox | |
URL | https://comptox.epa.gov/dashboard/DTXSID90583231 | |
Description | DSSTox provides a high quality public chemistry resource for supporting improved predictive toxicology. | |
Molecular Weight |
1225.4 g/mol | |
Source | PubChem | |
URL | https://pubchem.ncbi.nlm.nih.gov | |
Description | Data deposited in or computed by PubChem | |
CAS No. |
207739-72-8 | |
Record name | N~2~,N~2~,N~2'~,N~2'~,N~7~,N~7~,N~7'~,N~7'~-Octakis(4-methoxyphenyl)-9,9'-spirobi[fluorene]-2,2',7,7'-tetramine | |
Source | EPA DSSTox | |
URL | https://comptox.epa.gov/dashboard/DTXSID90583231 | |
Description | DSSTox provides a high quality public chemistry resource for supporting improved predictive toxicology. | |
Disclaimer and Information on In-Vitro Research Products
Please be aware that all articles and product information presented on BenchChem are intended solely for informational purposes. The products available for purchase on BenchChem are specifically designed for in-vitro studies, which are conducted outside of living organisms. In-vitro studies, derived from the Latin term "in glass," involve experiments performed in controlled laboratory settings using cells or tissues. It is important to note that these products are not categorized as medicines or drugs, and they have not received approval from the FDA for the prevention, treatment, or cure of any medical condition, ailment, or disease. We must emphasize that any form of bodily introduction of these products into humans or animals is strictly prohibited by law. It is essential to adhere to these guidelines to ensure compliance with legal and ethical standards in research and experimentation.