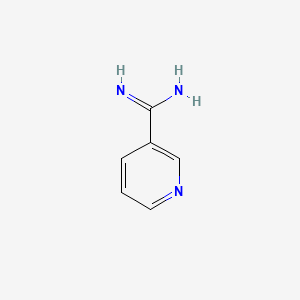
3-Pyridinecarboxamidine
Overview
Description
3-Pyridinecarboxamidine (CAS 7356-60-7), also known as 3-amidinopyridine hydrochloride, is a pyridine derivative with the molecular formula C₆H₈ClN₃ and a molecular weight of 157.60 g/mol . Its structure features a pyridine ring substituted at the 3-position with a carboxamidine group (–C(=NH)NH₂), which confers strong basicity and reactivity. This compound is soluble in polar solvents like water and dimethyl sulfoxide (DMSO) and is utilized in pharmaceutical research, particularly as a precursor for synthesizing antibacterial agents and enzyme inhibitors .
Preparation Methods
Synthetic Routes and Reaction Conditions: 3-Pyridinecarboxamidine is typically synthesized from 3-cyanopyridine. The process involves dissolving 3-cyanopyridine in dry chloroform and adding absolute ethyl alcohol. The mixture is cooled to 0°C while dry hydrogen chloride saturates the reaction mixture. After standing at 0°C for 16 hours, the mixture is poured into an ice-cold 50% sodium hydroxide solution to give an alkaline solution. The chloroform layer is separated, washed with water until neutral, dried over potassium carbonate, and concentrated to give the crude imidate. This imidate is then dissolved in 75% aqueous ethanol containing ammonium chloride and heated at 70°C for 4 hours. The product is obtained after filtration and dilution with acetone .
Industrial Production Methods: The industrial production of this compound follows similar synthetic routes but on a larger scale. The reaction conditions are optimized to ensure high yield and purity of the product. Safety measures are strictly followed to handle hazardous reagents and conditions .
Chemical Reactions Analysis
Types of Reactions: 3-Pyridinecarboxamidine undergoes various chemical reactions, including:
Oxidation: It can be oxidized to form corresponding oxides.
Reduction: It can be reduced to form amines.
Substitution: It can undergo substitution reactions with various electrophiles and nucleophiles.
Common Reagents and Conditions:
Oxidation: Common oxidizing agents include potassium permanganate and hydrogen peroxide.
Reduction: Reducing agents such as lithium aluminum hydride and sodium borohydride are used.
Substitution: Reagents like halogens, acids, and bases are commonly used.
Major Products Formed: The major products formed from these reactions depend on the specific reagents and conditions used. For example, oxidation may yield pyridine N-oxides, while reduction may produce pyridine amines .
Scientific Research Applications
Pharmaceutical Applications
1.1 Anti-Tubercular Activity
Recent studies have identified derivatives of 3-pyridinecarboxamidine as promising candidates in the fight against drug-resistant tuberculosis. One such derivative, MMV687254, has demonstrated potent anti-mycobacterial activity against Mycobacterium tuberculosis and Mycobacterium bovis Bacillus Calmette-Guérin (BCG). This compound acts in a bacteriostatic manner in liquid cultures and exhibits bactericidal effects within macrophages by inducing autophagy. The structure-activity relationship (SAR) studies have led to the identification of lead candidates that show efficacy in chronic mouse models of infection, highlighting the potential for developing new anti-tubercular agents based on this scaffold .
1.2 Dermatological Uses
This compound is widely recognized for its dermatological benefits. It is used in topical formulations to treat acne and improve skin appearance by reducing hyperpigmentation and fine lines associated with aging. Clinical trials have suggested that nicotinamide can significantly lower the incidence of nonmelanoma skin cancers among high-risk populations . Additionally, it has been evaluated for its role in treating various skin disorders such as psoriasis and rosacea .
1.3 Other Therapeutic Applications
The compound has shown potential in treating chronic kidney disease by reducing serum phosphorus levels in hemodialysis patients. It also exhibits anti-inflammatory properties that may benefit conditions like osteoarthritis by improving joint flexibility and reducing the need for anti-inflammatory medications . Furthermore, there is ongoing research into its efficacy for managing conditions like HIV and various autoimmune disorders .
Agricultural Applications
2.1 Disease Resistance in Plants
Research has explored the application of pyridine-3-carboxamide analogs in agriculture, particularly for combating bacterial wilt in tomatoes caused by Ralstonia solanacearum. These compounds have been shown to enhance plant growth and reduce infection rates significantly. Molecular docking studies indicate strong binding affinities between these compounds and bacterial proteins, suggesting their potential as effective agricultural treatments .
Compound | Target Pathogen | Effectiveness | Binding Energy (kcal/mol) |
---|---|---|---|
4a | Ralstonia solanacearum | High reduction in infection | -8.910 |
Cosmetic Applications
This compound is extensively utilized in cosmetic formulations due to its beneficial effects on skin health. It acts as a skin conditioning agent, enhancing the skin barrier function and providing anti-aging benefits . Its incorporation into creams and serums has been linked to improved hydration, elasticity, and overall skin appearance.
Research Insights and Case Studies
Numerous studies underscore the diverse applications of this compound:
Mechanism of Action
The mechanism by which 3-Pyridinecarboxamidine exerts its effects involves its interaction with molecular targets and pathways. For instance, it has been identified as a promising lead for Mycobacterium tuberculosis, where it inhibits bacterial growth by inducing autophagy in macrophages. The compound acts as a prodrug, requiring AmiC-dependent hydrolysis for its anti-mycobacterial activity .
Comparison with Similar Compounds
The following table summarizes key structural, physicochemical, and biological differences between 3-pyridinecarboxamidine and related pyridine derivatives:
Functional Group Analysis
- Carboxamidine vs. Carboxamide : The carboxamidine group in this compound is more basic than the carboxamide group in nicotinamide due to resonance stabilization of the amidinium ion. This enhances its ability to interact with negatively charged biological targets, such as bacterial enzymes .
- Thiosemicarbazone Derivatives : Pyridine-3-carbaldehyde thiosemicarbazones exhibit metal-chelating properties, which are absent in this compound. These compounds show broader antimicrobial activity but lower solubility in aqueous media .
- Positional Isomerism : 4-Pyridinecarboxamide derivatives (e.g., CAS 88329-47-9) differ in substitution position, leading to altered dipole moments and steric effects. This impacts their binding affinity to targets like kinases or receptors .
Physicochemical Properties
- Solubility : this compound’s solubility in polar solvents contrasts with the lipophilic nature of 4-pyridinecarboxamide derivatives, which are more soluble in dichloromethane .
- Stability : Oxime derivatives (e.g., pyridine-2-carboxamide oxime) are prone to hydrolysis under acidic conditions, whereas amidines are stable across a wider pH range .
Drug Development
- LY2033298 (a pyridinecarboxamide derivative) acts as a muscarinic acetylcholine receptor modulator, highlighting the role of pyridine scaffolds in central nervous system drugs .
- PF-01247324 (a trifluoromethyl-substituted pyridinecarboxamide) shows promise in pain management, emphasizing the impact of electron-withdrawing substituents on target selectivity .
Biological Activity
3-Pyridinecarboxamidine, also known as nicotinamide, is a compound with significant biological activity across various domains, including pharmacology and agriculture. This article explores its biological activities, mechanisms of action, and potential applications based on recent research findings.
Overview of Biological Activities
This compound exhibits a range of biological activities, including:
- Antimicrobial Properties : It has shown efficacy against various bacterial and fungal strains.
- Anti-inflammatory Effects : The compound has been noted for its ability to modulate inflammatory responses.
- Antitumor Activity : Research indicates potential in inhibiting tumor growth and promoting apoptosis in cancer cells.
- Urease Inhibition : Significant inhibition of urease activity has been documented, which is crucial for treating certain infections.
The biological effects of this compound can be attributed to several mechanisms:
- Enzyme Inhibition : The compound inhibits urease with varying potency depending on the substitution on the pyridine ring. For instance, derivatives showed IC50 values ranging from 1.07 µM to 14.49 µM against urease, indicating strong potential for therapeutic applications in managing urease-related conditions .
- Molecular Docking Studies : Molecular docking has revealed that this compound interacts with key amino acid residues in target proteins, facilitating strong hydrogen bonding and π–π interactions which enhance its biological activity .
- ADME Profile : Studies on absorption, distribution, metabolism, and excretion (ADME) suggest that the compound is well-absorbed and metabolized in vivo, contributing to its effectiveness .
Research Findings
Recent studies have further elucidated the biological activities of this compound:
- A study demonstrated that pyridine-3-carboxamide analogs significantly reduced bacterial wilt in tomatoes caused by Ralstonia solanacearum, showcasing their agricultural potential .
- Another investigation highlighted the anti-cancer properties of these compounds, indicating their role in inhibiting cell proliferation and inducing apoptosis in cancer cell lines .
Case Study 1: Urease Inhibition
A comparative study assessed the urease inhibitory activity of various pyridine carboxamide derivatives. The most potent derivative showed an IC50 value of 1.07 µM, which was significantly lower than other tested compounds. This finding underscores the potential use of these derivatives in treating infections caused by urease-producing pathogens.
Case Study 2: Antimicrobial Efficacy
In vitro tests revealed that this compound exhibited broad-spectrum antimicrobial activity against both Gram-positive and Gram-negative bacteria as well as certain fungi. The compound's mechanism appears linked to disrupting cellular processes essential for microbial survival.
Data Tables
Activity Type | IC50 Value (µM) | Notes |
---|---|---|
Urease Inhibition | 1.07 | Most potent derivative |
Antibacterial Activity | Varies | Effective against multiple strains |
Anti-inflammatory | N/A | Modulates cytokine release |
Antitumor Activity | N/A | Induces apoptosis in cancer cells |
Q & A
Basic Research Questions
Q. What are the common synthetic routes for 3-Pyridinecarboxamidine, and how can reaction conditions be optimized?
- Methodological Answer : Synthesis typically involves multi-step reactions, such as cyclization of intermediates or Rh-catalyzed tandem reactions. For example, pyridine core construction may require controlled temperature, solvent selection (e.g., DMF or THF), and catalyst optimization. Reaction progress should be monitored via TLC or HPLC. Adjusting pH and stoichiometric ratios of precursors (e.g., benzoyl derivatives) can enhance yield .
Q. Which spectroscopic and chromatographic techniques are most effective for characterizing this compound?
- Methodological Answer : Nuclear Magnetic Resonance (NMR) spectroscopy (¹H/¹³C) is critical for structural elucidation, particularly for confirming the amidine group. High-Resolution Mass Spectrometry (HRMS) validates molecular weight, while High-Performance Liquid Chromatography (HPLC) with UV detection ensures purity (>98%). Reference standards from databases like NIST Chemistry WebBook aid in spectral matching .
Q. How should researchers handle solubility challenges during in vitro assays?
- Methodological Answer : Solubility in aqueous buffers can be improved using co-solvents (e.g., DMSO ≤1% v/v) or surfactants (e.g., Tween-80). Pre-formulation studies, including dynamic light scattering (DLS) for particle size analysis, help identify aggregation issues. Solubility parameters (logP) calculated via computational tools (e.g., ChemAxon) guide solvent selection .
Advanced Research Questions
Q. How can structure-activity relationship (SAR) studies be designed to optimize this compound’s biological activity?
- Methodological Answer : Introduce functional groups (e.g., trifluoromethyl or halogen substituents) at positions 2, 4, or 6 of the pyridine ring to assess impact on target binding. Use parallel synthesis libraries and evaluate derivatives via enzyme inhibition assays (e.g., IC₅₀ determination). Molecular docking against crystallographic protein structures (PDB) identifies key interactions .
Q. What strategies resolve discrepancies in pharmacological data between in vitro and in vivo models?
- Methodological Answer : Cross-validate assays using orthogonal methods (e.g., SPR for binding affinity vs. cellular viability assays). Control for metabolic stability (e.g., liver microsome studies) and bioavailability differences. Apply the FINER criteria (Feasible, Novel, Ethical, Relevant) to refine experimental objectives and address confounding variables .
Q. How can computational modeling predict off-target effects of this compound derivatives?
- Methodological Answer : Perform molecular dynamics simulations to assess binding to non-target proteins (e.g., CYP450 isoforms). Use tools like SwissTargetPrediction or ChEMBL for polypharmacology profiling. Validate predictions with kinase panel screens or transcriptomic analyses (RNA-seq) .
Q. What safety protocols are critical when handling reactive intermediates during synthesis?
- Methodological Answer : Use fume hoods for volatile intermediates (e.g., chlorinated precursors). Monitor exothermic reactions with temperature probes and employ quenching protocols (e.g., ice baths for acyl chlorides). Safety Data Sheets (SDS) for analogs (e.g., 3-benzoyl derivatives) provide hazard benchmarks .
Q. Data Analysis and Experimental Design
Q. How do researchers troubleshoot low yields in amidine functionalization reactions?
- Methodological Answer : Evaluate catalyst efficiency (e.g., Pd/C vs. Rh complexes) and moisture sensitivity of reagents. Use inert atmospheres (argon/glovebox) for moisture-sensitive steps. Kinetic studies (e.g., varying reaction time) identify rate-limiting steps. Alternative activating agents (e.g., EDCI/HOBt) may improve coupling efficiency .
Q. What statistical approaches are recommended for analyzing dose-response data in toxicity studies?
- Methodological Answer : Apply nonlinear regression models (e.g., sigmoidal dose-response curves) to calculate LD₅₀/LC₅₀. Use ANOVA with post-hoc Tukey tests to compare treatment groups. Survival analysis (Kaplan-Meier curves) assesses time-dependent effects. Open-source tools like R/Bioconductor ensure reproducibility .
Q. How can interdisciplinary approaches enhance this compound’s application in drug discovery?
- Methodological Answer : Integrate medicinal chemistry with cheminformatics (e.g., QSAR models) and bioinformatics (e.g., pathway enrichment analysis). Collaborate with crystallography labs for target-ligand co-structures. Validate findings in disease-relevant models (e.g., patient-derived organoids) .
Properties
IUPAC Name |
pyridine-3-carboximidamide | |
---|---|---|
Details | Computed by Lexichem TK 2.7.0 (PubChem release 2021.05.07) | |
Source | PubChem | |
URL | https://pubchem.ncbi.nlm.nih.gov | |
Description | Data deposited in or computed by PubChem | |
InChI |
InChI=1S/C6H7N3/c7-6(8)5-2-1-3-9-4-5/h1-4H,(H3,7,8) | |
Details | Computed by InChI 1.0.6 (PubChem release 2021.05.07) | |
Source | PubChem | |
URL | https://pubchem.ncbi.nlm.nih.gov | |
Description | Data deposited in or computed by PubChem | |
InChI Key |
FVGUUJNEJJPLCS-UHFFFAOYSA-N | |
Details | Computed by InChI 1.0.6 (PubChem release 2021.05.07) | |
Source | PubChem | |
URL | https://pubchem.ncbi.nlm.nih.gov | |
Description | Data deposited in or computed by PubChem | |
Canonical SMILES |
C1=CC(=CN=C1)C(=N)N | |
Details | Computed by OEChem 2.3.0 (PubChem release 2021.05.07) | |
Source | PubChem | |
URL | https://pubchem.ncbi.nlm.nih.gov | |
Description | Data deposited in or computed by PubChem | |
Molecular Formula |
C6H7N3 | |
Details | Computed by PubChem 2.1 (PubChem release 2021.05.07) | |
Source | PubChem | |
URL | https://pubchem.ncbi.nlm.nih.gov | |
Description | Data deposited in or computed by PubChem | |
DSSTOX Substance ID |
DTXSID40945941 | |
Record name | Pyridine-3-carboximidamide | |
Source | EPA DSSTox | |
URL | https://comptox.epa.gov/dashboard/DTXSID40945941 | |
Description | DSSTox provides a high quality public chemistry resource for supporting improved predictive toxicology. | |
Molecular Weight |
121.14 g/mol | |
Details | Computed by PubChem 2.1 (PubChem release 2021.05.07) | |
Source | PubChem | |
URL | https://pubchem.ncbi.nlm.nih.gov | |
Description | Data deposited in or computed by PubChem | |
CAS No. |
23255-20-1 | |
Record name | Pyridine-3-carboximidamide | |
Source | EPA DSSTox | |
URL | https://comptox.epa.gov/dashboard/DTXSID40945941 | |
Description | DSSTox provides a high quality public chemistry resource for supporting improved predictive toxicology. | |
Disclaimer and Information on In-Vitro Research Products
Please be aware that all articles and product information presented on BenchChem are intended solely for informational purposes. The products available for purchase on BenchChem are specifically designed for in-vitro studies, which are conducted outside of living organisms. In-vitro studies, derived from the Latin term "in glass," involve experiments performed in controlled laboratory settings using cells or tissues. It is important to note that these products are not categorized as medicines or drugs, and they have not received approval from the FDA for the prevention, treatment, or cure of any medical condition, ailment, or disease. We must emphasize that any form of bodily introduction of these products into humans or animals is strictly prohibited by law. It is essential to adhere to these guidelines to ensure compliance with legal and ethical standards in research and experimentation.