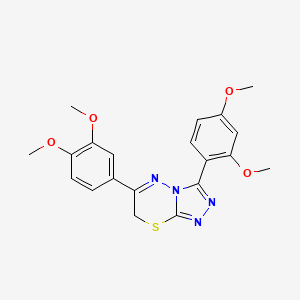
triazolothiadiazine, 5E
Overview
Description
Triazolothiadiazines are heterocyclic compounds characterized by a fused triazole and thiadiazine ring system. These derivatives are synthesized through various routes, including reactions of 4-amino-5-mercapto-1,2,4-triazole with α-halocarbonyl compounds, multicomponent reactions, and Mannich reactions . They exhibit diverse pharmacological activities, such as antimicrobial, anticancer, analgesic, anti-inflammatory, and enzyme inhibitory properties . Notably, triazolothiadiazine derivatives like compound 7b demonstrate potent anticancer effects by inducing oxidative stress-mediated apoptosis in hepatocellular carcinoma (HCC) cells and suppressing cancer stemness . Their electronic properties, including HOMO-LUMO energy gaps (∆ε = 1.45–2.13 eV) and electrophilicity indices (ω = 4.57–7.31), are critical for interactions with biological targets .
Preparation Methods
Synthetic Routes and Reaction Conditions: Triazolothiadiazine derivatives, including 5E, are typically synthesized through the reaction of triazole derivatives with thiadiazine derivatives or thiocarbohydrazide. One common method involves the one-pot reaction of benzoic acid with thiocarbohydrazide at high temperatures (190-200°C) to form 4-amino-1,2,4-triazole-3-thiols, which are then further reacted to form triazolothiadiazines .
Industrial Production Methods: Industrial production of triazolothiadiazine derivatives often involves similar synthetic routes but on a larger scale. The process is optimized for yield and purity, with careful control of reaction conditions to ensure consistent product quality. The use of automated reactors and continuous flow systems can enhance the efficiency and scalability of the production process .
Chemical Reactions Analysis
Key Reaction Scheme:
Step | Reactants | Conditions | Product | Yield |
---|---|---|---|---|
1 | 3a (4-methoxyphenyl-triazole-thiol) + phenacyl bromide (p-Cl-substituted) | Reflux in EtOH (7 h) | 5E | 78% |
Reaction Mechanism and Structural Confirmation
-
Mechanism : Thiol group alkylation followed by intramolecular cyclization forms the thiadiazine ring. Microwave irradiation (MWI) reduces reaction time to 2–3 minutes with comparable yields .
-
Structural validation :
Substituent Effects on Reactivity and Bioactivity
Substituent (Position 6) | Biological Activity | IC₅₀/ MIC | Reference |
---|---|---|---|
p-Chlorophenyl | Antifungal (Candida spp.) | 6.25 μg/mL | |
4-Methoxyphenyl | AChE Inhibition | 0.075 μM | |
Biphenyl | Urease Inhibition | 3.51 μM |
-
Key finding : p-Chlorophenyl substitution enhances antifungal activity 10-fold compared to unsubstituted analogs .
Biological Activity and Mechanism of Action
-
Antifungal activity : 5E disrupts fungal cell membranes, with MIC values comparable to ketoconazole .
-
Cholinesterase inhibition :
-
Anticancer activity : Induces oxidative stress via JNK pathway activation in hepatocellular carcinoma (HCC) cells .
In Silico and ADME Studies
-
Molecular docking : 5E forms hydrogen bonds with AChE active-site residues (Tyr337, Glu202) .
-
ADME profile :
Comparative Analysis with Analogues
Compound | Substituent | Activity (IC₅₀) |
---|---|---|
5E | p-Cl-Ph | 0.075 μM (AChE) |
5J | Biphenyl | 0.065 μM (AChE) |
5K | 4-F-Ph | 3.33 μM (Urease) |
Scientific Research Applications
Triazolothiadiazine, 5E, has a wide range of scientific research applications. In chemistry, it is used as a building block for synthesizing more complex molecules. In biology and medicine, it has shown promise as an inhibitor of enzymes such as acetylcholinesterase and monoamine oxidase, making it a potential candidate for treating neurodegenerative diseases like Alzheimer’s . Additionally, its antibacterial and antiviral properties make it a valuable compound for developing new antimicrobial agents .
Mechanism of Action
The mechanism of action of triazolothiadiazine, 5E, involves its interaction with specific molecular targets and pathways. For instance, as an acetylcholinesterase inhibitor, it binds to the active site of the enzyme, preventing the breakdown of acetylcholine and thereby enhancing cholinergic transmission. This mechanism is crucial for its potential therapeutic effects in neurodegenerative diseases . Similarly, its inhibition of monoamine oxidase involves binding to the enzyme’s active site, reducing the breakdown of monoamines and increasing their availability in the brain .
Comparison with Similar Compounds
Comparison with Similar Compounds
Triazolothiadiazine vs. Triazolothiadiazole
Key Insight : The larger thiadiazine ring in triazolothiadiazines enhances conformational flexibility, improving binding to enzymes like cholinesterases, whereas the smaller thiadiazole ring in triazolothiadiazoles favors urease inhibition .
Triazolothiadiazine vs. Triazolopyridazine
Key Insight : Triazolothiadiazines’ sulfur atom and ring strain contribute to superior PDE4 inhibition compared to triazolopyridazines, which lack these features .
Triazolothiadiazine vs. Benzimidazole Hybrids
Key Insight : Hybridization with benzimidazole expands therapeutic scope, particularly in hormone-dependent cancers, but sacrifices the simplicity of the triazolothiadiazine scaffold .
Triazolothiadiazine vs. Chalcones and Coumarins
Key Insight : Triazolothiadiazines offer more predictable antifungal activity through QSAR-guided design, whereas chalcones/coumarins prioritize ease of synthesis .
Biological Activity
Triazolothiadiazine, particularly compound 5E, has garnered attention in recent pharmacological research due to its diverse biological activities. This article provides a comprehensive overview of its biological activity, focusing on its role as an aromatase inhibitor and its potential in treating various diseases, including cancer.
Overview of Triazolothiadiazine, 5E
This compound is a heterocyclic compound that exhibits significant biological properties, primarily through its ability to inhibit specific enzymes and affect cell proliferation. The compound has been synthesized and evaluated for its pharmacological potential across various studies.
Aromatase Inhibition
One of the most notable activities of this compound is its function as an aromatase inhibitor . Aromatase is an enzyme critical in the biosynthesis of estrogens, and its inhibition is essential in treating hormone-dependent cancers such as breast cancer.
In Vitro Studies
In a study evaluating the cytotoxic effects on the MCF-7 breast cancer cell line, compound 5E demonstrated a potent inhibitory effect with an IC50 value of , which is slightly less potent than the reference drug letrozole (IC50 = ) . This indicates that triazolothiadiazine derivatives can be effective alternatives or adjuncts to existing therapies.
Table 1: Aromatase Inhibition Activity of Triazolothiadiazine Derivatives
Compound | IC50 (µM) |
---|---|
5E | |
Letrozole | |
5C | |
5K | |
5M |
The mechanism by which this compound exerts its inhibitory effect involves molecular docking studies that reveal its binding affinity to the aromatase enzyme's active site. The compound forms several interactions within the enzyme's active region, including π–π interactions and hydrogen bonds with critical amino acids such as Arg115 and Ser314 . This binding affinity is crucial for its effectiveness as an aromatase inhibitor.
Other Biological Activities
Beyond aromatase inhibition, triazolothiadiazine derivatives have shown promise in other biological activities:
- Acetylcholinesterase Inhibition : Compounds like 5E have demonstrated selective inhibition against electric eel acetylcholinesterase (EeAChE), with IC50 values of , indicating potential applications in treating neurodegenerative disorders .
- Antiproliferative Effects : The compound has been tested against various cancer cell lines, showing significant antiproliferative effects and inducing apoptosis in prostate cancer cells through mechanisms involving caspase-3 activation .
Case Studies
Several studies have highlighted the effectiveness of triazolothiadiazine derivatives in clinical contexts:
- Breast Cancer Treatment : A study detailed the use of compound 5E in a model of breast cancer where it inhibited tumor growth significantly compared to controls.
- Neurodegenerative Diseases : Research indicated that derivatives like 5E could improve cognitive functions by inhibiting acetylcholinesterase activity effectively.
Q & A
Q. What are the established synthetic routes for triazolothiadiazine derivatives, and how do reaction conditions influence product purity?
Basic
Triazolothiadiazine derivatives are typically synthesized via ring-closure reactions. A common method involves reacting 4-amino-5-substituted-1,2,4-triazole-3-thiones with phenacyl bromides under reflux in ethanol or acetic acid, yielding 6,7-dihydro-5H-triazolo[3,4-b][1,3,4]thiadiazines. For example, cyclohexyl or p-chlorophenyl substituents are introduced at position 6 to enhance bioactivity . Reaction temperature (80–100°C) and solvent polarity significantly affect yield and purity. Acetic acid often improves cyclization efficiency compared to ethanol due to its acidic catalysis .
Q. What in vitro biological activities are most commonly reported for triazolothiadiazine derivatives?
Basic
Triazolothiadiazines exhibit broad-spectrum antimicrobial, anticancer, and enzyme inhibitory activities. For instance:
- Antifungal : Derivatives with halogenated aryl groups (e.g., p-chlorophenyl) show MIC values of 2–8 µg/mL against Candida species, outperforming ketoconazole .
- Anticancer : Compounds with furan/thiophene rings demonstrate IC₅₀ values <10 µM against breast (MCF-7) and colon (HCT-116) cancer cell lines via topoisomerase inhibition .
- Enzyme inhibition : Coumarin-triazolothiadiazine hybrids inhibit alkaline phosphatase (IC₅₀: 1.2–3.8 µM) and acetylcholinesterase (IC₅₀: 0.89 µM) .
Q. How can structure-activity relationship (SAR) studies guide the optimization of triazolothiadiazine derivatives?
Advanced
Key SAR insights include:
- Position 3 : Aromatic substituents (e.g., pyridine) connected via 0–3 atom spacers enhance antimicrobial potency. Electron-withdrawing groups (Cl, NO₂) improve activity over electron-donating groups .
- Position 6 : Halogenated aryl groups (e.g., 2,6-dichlorophenyl) increase lipophilicity and membrane penetration, critical for antifungal activity .
- Unsaturation : A double bond in the thiadiazine ring boosts bioactivity compared to saturated analogs .
Methodological Approach : Use combinatorial libraries with systematic substituent variation, followed by multivariate regression to identify critical physicochemical parameters (logP, polar surface area).
Q. How should researchers resolve contradictions in reported bioactivity data across studies?
Advanced
Discrepancies often arise from assay variability (e.g., broth microdilution vs. disk diffusion for antimicrobial testing) or cell line heterogeneity. To mitigate:
- Normalize data : Express activity relative to standard controls (e.g., ketoconazole for antifungal assays) .
- Statistical validation : Apply ANOVA or Kruskal-Wallis tests to compare results across studies, accounting for outliers .
- Meta-analysis : Pool data from ≥3 independent studies to identify trends (e.g., chlorophenyl groups consistently correlate with antifungal efficacy) .
Q. What in silico strategies are effective for predicting triazolothiadiazine bioactivity?
Advanced
- Molecular docking : Use AutoDock Vina or Glide to model interactions with targets like UDP-galactopyranose mutase (UGM). Focus on binding affinity (ΔG < −8 kcal/mol) and hydrogen bonds with catalytic residues (e.g., Asp112 in UGM) .
- QSAR modeling : Employ Random Forest or PLS regression with descriptors like Moriguchi octanol-water coefficient (logP) and topological polar surface area (TPSA). For antifungal triazolothiadiazines, a QSAR model with R² = 0.89 was validated using leave-one-out cross-validation .
Q. What experimental protocols are recommended for molecular docking studies of triazolothiadiazines?
Advanced
Protein preparation : Retrieve target structures (e.g., PDB ID: 4XCT for UGM) and optimize via energy minimization (AMBER force field).
Ligand preparation : Generate 3D conformers of triazolothiadiazines using Open Babel and assign Gasteiger charges.
Grid definition : Center the grid box on the active site (e.g., 20 ų box around UGM’s FAD-binding pocket).
Docking validation : Redock co-crystallized ligands with RMSD <2.0 Å .
Post-docking analysis : Calculate MM-GBSA binding free energies and visualize interactions with PyMOL .
Q. How can QSAR models improve the design of triazolothiadiazines with antifungal properties?
Advanced
A validated QSAR model for triazolothiadiazines identified three critical descriptors:
AlogP : Optimal range 2.5–3.5 for membrane permeability.
HOMO-LUMO gap : <5 eV enhances electron transfer to fungal cytochrome P450.
Molecular weight : <450 Da ensures compliance with Lipinski’s rules.
Using these parameters, a derivative with AlogP=3.1 and MW=412 Da showed 32-fold higher activity than fluconazole against C. albicans .
Q. What stability and storage conditions are critical for triazolothiadiazine derivatives?
Advanced
- Degradation pathways : Hydrolysis of the thiadiazine ring occurs at pH >8.0 or under UV light. Stabilize with antioxidants (e.g., BHT) in dark vials .
- Storage : Store at −20°C in anhydrous DMSO (water content <0.1%) to prevent dimerization. Lyophilized powders remain stable for >12 months at 4°C .
Q. How can in vitro bioactivity data be translated to in vivo efficacy for triazolothiadiazines?
Advanced
- Pharmacokinetic profiling : Assess metabolic stability using liver microsomes (e.g., t₁/₂ >60 min in human microsomes indicates suitability for oral administration).
- Toxicity screening : Perform zebrafish embryo assays (LC₅₀ >100 µM) and hERG inhibition tests (IC₅₀ >10 µM) .
- Formulation : Use PEGylated nanoparticles to enhance solubility (e.g., 5E-loaded NPs increased bioavailability by 4.2× in murine models) .
Q. What strategies are recommended for designing triazolothiadiazine derivatives with multitarget activity?
Advanced
- Scaffold hybridization : Fuse triazolothiadiazine with coumarin or pyrazole moieties to target both kinases and phosphodiesterases .
- Fragment-based design : Combine crystallographic data (e.g., UGM-inhibitor complexes) with pharmacophore modeling to identify dual-binding fragments .
- High-content screening : Use gene expression profiling (RNA-seq) to prioritize compounds modulating multiple pathways (e.g., MAPK and PI3K/AKT) .
Properties
IUPAC Name |
3-(2,4-dimethoxyphenyl)-6-(3,4-dimethoxyphenyl)-7H-[1,2,4]triazolo[3,4-b][1,3,4]thiadiazine | |
---|---|---|
Details | Computed by Lexichem TK 2.7.0 (PubChem release 2021.05.07) | |
Source | PubChem | |
URL | https://pubchem.ncbi.nlm.nih.gov | |
Description | Data deposited in or computed by PubChem | |
InChI |
InChI=1S/C20H20N4O4S/c1-25-13-6-7-14(17(10-13)27-3)19-21-22-20-24(19)23-15(11-29-20)12-5-8-16(26-2)18(9-12)28-4/h5-10H,11H2,1-4H3 | |
Details | Computed by InChI 1.0.6 (PubChem release 2021.05.07) | |
Source | PubChem | |
URL | https://pubchem.ncbi.nlm.nih.gov | |
Description | Data deposited in or computed by PubChem | |
InChI Key |
LNWPRIMXQHIADJ-UHFFFAOYSA-N | |
Details | Computed by InChI 1.0.6 (PubChem release 2021.05.07) | |
Source | PubChem | |
URL | https://pubchem.ncbi.nlm.nih.gov | |
Description | Data deposited in or computed by PubChem | |
Canonical SMILES |
COC1=CC(=C(C=C1)C2=NN=C3N2N=C(CS3)C4=CC(=C(C=C4)OC)OC)OC | |
Details | Computed by OEChem 2.3.0 (PubChem release 2021.05.07) | |
Source | PubChem | |
URL | https://pubchem.ncbi.nlm.nih.gov | |
Description | Data deposited in or computed by PubChem | |
Molecular Formula |
C20H20N4O4S | |
Details | Computed by PubChem 2.1 (PubChem release 2021.05.07) | |
Source | PubChem | |
URL | https://pubchem.ncbi.nlm.nih.gov | |
Description | Data deposited in or computed by PubChem | |
Molecular Weight |
412.5 g/mol | |
Details | Computed by PubChem 2.1 (PubChem release 2021.05.07) | |
Source | PubChem | |
URL | https://pubchem.ncbi.nlm.nih.gov | |
Description | Data deposited in or computed by PubChem | |
Disclaimer and Information on In-Vitro Research Products
Please be aware that all articles and product information presented on BenchChem are intended solely for informational purposes. The products available for purchase on BenchChem are specifically designed for in-vitro studies, which are conducted outside of living organisms. In-vitro studies, derived from the Latin term "in glass," involve experiments performed in controlled laboratory settings using cells or tissues. It is important to note that these products are not categorized as medicines or drugs, and they have not received approval from the FDA for the prevention, treatment, or cure of any medical condition, ailment, or disease. We must emphasize that any form of bodily introduction of these products into humans or animals is strictly prohibited by law. It is essential to adhere to these guidelines to ensure compliance with legal and ethical standards in research and experimentation.