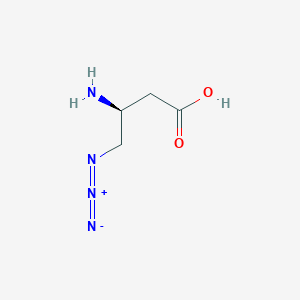
(3S)-3-amino-4-azidobutanoic acid
- Click on QUICK INQUIRY to receive a quote from our team of experts.
- With the quality product at a COMPETITIVE price, you can focus more on your research.
Overview
Description
(3S)-3-amino-4-azidobutanoic acid, also known as AABA, is a non-proteinogenic amino acid that contains an azide group (-N3) and an amino group (-NH2) in its chemical structure. It has gained significant attention in the scientific community due to its unique properties and potential applications in various fields, including biochemistry, molecular biology, and medicinal chemistry.
Mechanism of Action
(3S)-3-amino-4-azidobutanoic acid works by reacting with specific amino acid residues in proteins and peptides, such as cysteine or lysine, through a process known as azide-alkyne cycloaddition. This reaction forms a covalent bond between this compound and the target amino acid residue, which can then be detected using various analytical techniques.
Biochemical and physiological effects:
This compound has been shown to have minimal effects on the biochemical and physiological properties of proteins and peptides when used in low concentrations. However, at higher concentrations, this compound can potentially affect the activity and stability of these molecules, which may limit its use in certain applications.
Advantages and Limitations for Lab Experiments
One of the main advantages of using (3S)-3-amino-4-azidobutanoic acid in lab experiments is its ability to selectively label specific amino acid residues in proteins and peptides, which allows for precise identification and characterization of these molecules. Additionally, this compound is relatively easy to synthesize and can be produced in high yields and purity.
However, there are also some limitations to using this compound in lab experiments. One of the main limitations is its potential to affect the activity and stability of proteins and peptides at higher concentrations. Additionally, the use of this compound requires specialized analytical techniques, which may limit its accessibility to some researchers.
Future Directions
There are several potential future directions for the use of (3S)-3-amino-4-azidobutanoic acid in scientific research. One area of interest is the development of new methods for site-specific labeling of proteins and peptides using this compound. Additionally, there is potential for the use of this compound in the study of protein-protein interactions and the development of new crosslinking agents for the study of protein structure and function.
In conclusion, this compound is a unique and versatile amino acid that has gained significant attention in the scientific community due to its potential applications in various fields. Its ability to selectively label specific amino acid residues in proteins and peptides makes it a valuable tool for the identification and characterization of these molecules. However, its potential effects on the activity and stability of proteins and peptides at higher concentrations may limit its use in certain applications. Nonetheless, the future looks promising for the continued exploration of this compound's potential in scientific research.
Synthesis Methods
(3S)-3-amino-4-azidobutanoic acid can be synthesized using several methods, including the reaction of 3-bromo-4-chlorobutanenitrile with sodium azide and subsequent hydrolysis, or the reaction of 3-bromo-4-chlorobutanoic acid with sodium azide and subsequent reduction. These methods have been well-established and have been used to produce this compound in high yields and purity.
Scientific Research Applications
(3S)-3-amino-4-azidobutanoic acid has been extensively studied for its potential applications in scientific research. It has been used as a tool for site-specific labeling of proteins and peptides, which allows for the identification and characterization of specific amino acid residues in these molecules. This compound has also been used as a photoaffinity probe for the identification of protein-protein interactions and as a crosslinking agent for the study of protein structure and function.
properties
IUPAC Name |
(3S)-3-amino-4-azidobutanoic acid |
Source
|
---|---|---|
Details | Computed by LexiChem 2.6.6 (PubChem release 2019.06.18) | |
Source | PubChem | |
URL | https://pubchem.ncbi.nlm.nih.gov | |
Description | Data deposited in or computed by PubChem | |
InChI |
InChI=1S/C4H8N4O2/c5-3(1-4(9)10)2-7-8-6/h3H,1-2,5H2,(H,9,10)/t3-/m0/s1 |
Source
|
Details | Computed by InChI 1.0.5 (PubChem release 2019.06.18) | |
Source | PubChem | |
URL | https://pubchem.ncbi.nlm.nih.gov | |
Description | Data deposited in or computed by PubChem | |
InChI Key |
JRKNECXMMGNWMM-VKHMYHEASA-N |
Source
|
Details | Computed by InChI 1.0.5 (PubChem release 2019.06.18) | |
Source | PubChem | |
URL | https://pubchem.ncbi.nlm.nih.gov | |
Description | Data deposited in or computed by PubChem | |
Canonical SMILES |
C(C(CN=[N+]=[N-])N)C(=O)O |
Source
|
Details | Computed by OEChem 2.1.5 (PubChem release 2019.06.18) | |
Source | PubChem | |
URL | https://pubchem.ncbi.nlm.nih.gov | |
Description | Data deposited in or computed by PubChem | |
Isomeric SMILES |
C([C@@H](CN=[N+]=[N-])N)C(=O)O |
Source
|
Details | Computed by OEChem 2.1.5 (PubChem release 2019.06.18) | |
Source | PubChem | |
URL | https://pubchem.ncbi.nlm.nih.gov | |
Description | Data deposited in or computed by PubChem | |
Molecular Formula |
C4H8N4O2 |
Source
|
Details | Computed by PubChem 2.1 (PubChem release 2019.06.18) | |
Source | PubChem | |
URL | https://pubchem.ncbi.nlm.nih.gov | |
Description | Data deposited in or computed by PubChem | |
Molecular Weight |
144.13 g/mol |
Source
|
Details | Computed by PubChem 2.1 (PubChem release 2021.05.07) | |
Source | PubChem | |
URL | https://pubchem.ncbi.nlm.nih.gov | |
Description | Data deposited in or computed by PubChem | |
Disclaimer and Information on In-Vitro Research Products
Please be aware that all articles and product information presented on BenchChem are intended solely for informational purposes. The products available for purchase on BenchChem are specifically designed for in-vitro studies, which are conducted outside of living organisms. In-vitro studies, derived from the Latin term "in glass," involve experiments performed in controlled laboratory settings using cells or tissues. It is important to note that these products are not categorized as medicines or drugs, and they have not received approval from the FDA for the prevention, treatment, or cure of any medical condition, ailment, or disease. We must emphasize that any form of bodily introduction of these products into humans or animals is strictly prohibited by law. It is essential to adhere to these guidelines to ensure compliance with legal and ethical standards in research and experimentation.