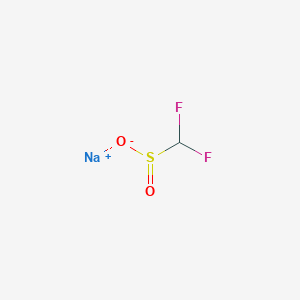
sodium difluoromethanesulfinate
Overview
Description
Sodium difluoromethanesulfinate (NaSO₂CF₂H, CAS 275818-95-6) is a versatile fluorinated sulfinate salt widely employed as a difluoromethyl (*CF₂H) radical precursor in organic synthesis. Its unique reactivity stems from the sulfinate group (SO₂⁻), which facilitates homolytic cleavage under oxidative or photochemical conditions to generate *CF₂H radicals .
Preparation Methods
Synthetic Routes and Reaction Conditions: Sodium difluoromethanesulfinate can be synthesized through several methods. One common approach involves the reaction of difluoromethyl sulfone with sodium hydroxide. Another method includes the electrochemical oxidation of difluoromethylated indoles under catalyst- and oxidant-free conditions . This green and efficient method uses this compound as the fluorinating reagent, yielding various indole derivatives with difluoromethylation at the C-2 position.
Industrial Production Methods: Industrial production of this compound typically involves large-scale chemical reactions under controlled conditions. The process often includes the use of specialized equipment to ensure the purity and yield of the final product. The reaction conditions are optimized to achieve high efficiency and minimal by-products .
Chemical Reactions Analysis
Radical Fluoroalkylation Reactions
Sodium difluoromethanesulfinate serves as a robust CF₂H radical source under oxidative conditions. Key findings include:
Reactivity with Conjugated N-Arylsulfonylated Amides
-
Mechanism : CF₂H radicals generated via AgNO₃/K₂S₂O₈-mediated oxidation undergo fluoroalkylative aryl migration, forming α-aryl-β-difluoromethyl amides (e.g., 4a ) (Table 1) .
-
Optimal Conditions :
-
Reactivity Order : CF₂PhSO₂Na (2b ) > CF₂HSO₂Na (2a ) > CH₂FSO₂Na (2c ) due to radical stability and steric effects .
Multicomponent Reactions
A visible-light-induced protocol enables the synthesis of CF₂H-substituted secondary amines:
-
Reaction : Aromatic aldehydes + amines + HCF₂SO₂Na → CF₂H-Secondary Amines .
-
Conditions : Room temperature, visible light (e.g., blue LED), 16–24 h.
-
Scope : Yields range from 43% to 79%, tolerating electron-donating and withdrawing groups on aldehydes .
Electrochemical Difluoromethylation
Electrochemical methods enable regioselective functionalization under mild conditions:
-
N-ortho-Selective Difluoromethylation :
-
Mechanism : Radical addition followed by hydrogen elimination (supported by radical-trapping experiments) .
Nucleophilic Substitution Reactions
HCF₂SO₂Na participates in S–C bond-forming reactions:
-
Thiol Difluoromethylation :
Photoinduced Difluoromethylation
Eosin Y-catalyzed reactions under aerobic conditions:
-
Mechanism : Single-electron transfer (SET) generates CF₂H radicals, which add to electron-deficient positions .
Comparative Reaction Data
Mechanistic Insights
-
Radical Generation : Ag⁺ or peroxydisulfate (S₂O₈²⁻) oxidizes HCF₂SO₂Na to CF₂H radicals .
-
Solvent Effects : Polar aprotic solvents (e.g., DMSO, NMP) enhance radical stability and reaction efficiency .
-
Competing Pathways : Alkyl substrates favor cyclization, while aryl groups promote desulfonylation .
Scientific Research Applications
Sodium difluoromethanesulfinate is a versatile reagent with diverse applications in chemical synthesis, particularly in the introduction of difluoromethyl groups into organic molecules . This compound has gained prominence as a building block for creating valuable organosulfur compounds and in late-stage difluoromethylation reactions .
Synthesis of Organosulfur Compounds
Sodium sulfinates, including this compound, serve as versatile building blocks in synthesizing various organosulfur compounds through reactions that form S–S, N–S, and C–S bonds . These reactions are used in the synthesis of thiosulfonates, sulfonamides, sulfides, and sulfones, including vinyl sulfones, allyl sulfones, and β-keto sulfones . Sodium sulfinates can act as sulfonylating, sulfenylating, or sulfinylating reagents, depending on the specific reaction conditions .
Concepts and Developments
Late-stage difluoromethylation is a chemical modification process where a difluoromethyl (CF2H) group is added to a molecule in the later stages of its synthesis . This is particularly useful in drug discovery and development because the introduction of a difluoromethyl group can modulate the lipophilicity and alter the ADME (absorption, distribution, metabolism, and excretion) properties of a drug candidate .
Lipophilicity Modulation
The difluoromethyl group can impact lipophilicity. For example, difluoromethylbenzene is more lipophilic than phenol, and difluoromethyl phenyl sulfide is more lipophilic than thiophenol . Studies have shown that ArOCF2H compounds (compounds with a difluoromethyl group bonded to an arene through an oxygen atom) tend to display decreased lipophilicity but higher permeability compared to ArOCF3 analogs .
Reagent Activation
Difluoromethyl radicals can be generated from this compound when activated by Eosin Y, using air as a stoichiometric oxidant .
C–H Functionalization of Heterocycles
Sodium trifluoromethanesulfinate can trifluoromethylate heterocycles . For example, it can be used in the trifluoromethylation of pentoxifylline .
Tetrafluoroisopropylation of Alkenes and Alkynes
This compound can serve as a source of the tetrafluoroisopropyl group via visible light photocatalysis .
Reaction Conditions and Yields
The yield of reactions involving this compound can vary based on reaction conditions . For instance, a reaction using dipotassium peroxodisulfate and silver nitrate in water and acetonitrile at 80°C for 24 hours resulted in a 61% yield .
Photoredox Catalysis
Mechanism of Action
The mechanism of action of sodium difluoromethanesulfinate involves the generation of difluoromethyl radicals. These radicals can react with various substrates, leading to the formation of difluoromethylated products. The difluoromethyl group acts as a lipophilic hydrogen bond donor, which enhances membrane permeability and improves the bioavailability of the resulting compounds . The high electronegativity of fluorine also affects the lipophilicity profile and inhibits certain metabolic pathways at the molecular level .
Comparison with Similar Compounds
Mechanistic Insights
The *CF₂H radical generated from NaSO₂CF₂H exhibits nucleophilic character due to the electron-donating fluorine atoms, enabling selective addition to electron-deficient heterocycles. This contrasts with electrophilic *CF₃ radicals, which require electron-rich substrates .
Comparison with Similar Sulfinate Compounds
Sodium sulfinates vary in reactivity based on substituents (alkyl, aryl, fluoroalkyl). Below is a comparative analysis of NaSO₂CF₂H with structurally related compounds.
Reactivity and Substrate Compatibility
Reaction Conditions and Efficiency
- NaSO₂CF₂H vs. Zinc Sulfinates: DFMS (Zn(SO₂CF₂H)₂) outperforms NaSO₂CF₂H in radical reactions due to lower oxidation potentials (+1.35 V vs. SCE) and compatibility with milder conditions (e.g., visible light, room temperature) .
- Electrochemical Applications : NaSO₂CF₂H is effective in nickel-catalyzed electrochemical difluoromethylation of aryl iodides but fails with aryl bromides .
- Photoredox Catalysis : NaSO₂CF₂H pairs with organic dyes (e.g., eosin Y) for metal-free difluoromethylation, whereas sodium triflate requires Ir/Ru photocatalysts .
Substrate-Specific Performance
- Scalability : NaSO₂CF₂H-based electrochemical methods are scalable (up to 10 mmol) with yields >80% .
Biological Activity
Sodium difluoromethanesulfinate (NaSO₂CF₂H) is a fluorinated sulfonate compound that has gained attention for its diverse biological activities and applications in organic synthesis. This article provides a comprehensive overview of its biological activity, including mechanisms, case studies, and relevant research findings.
This compound acts primarily as a source of the difluoromethyl radical (CF₂H- ) upon activation. This radical can participate in various reactions, leading to the formation of difluoromethylated products. The generation of CF₂H- can occur through different methods, including electrochemical oxidation and photoredox catalysis.
- Electrochemical Activation : this compound can be oxidized anodically to produce the difluoroalkyl radical, which is crucial in many synthetic transformations .
- Photoredox Catalysis : Under light irradiation, this compound can yield CF₂H- through a single-electron transfer mechanism, facilitating the formation of difluoromethylated compounds in mild conditions .
Biological Applications
The biological activity of this compound is primarily explored in the context of medicinal chemistry and agrochemicals. Its unique properties allow it to serve as a versatile building block for drug development.
- Antimicrobial Activity : Compounds containing difluoromethyl groups have shown enhanced antimicrobial properties. For instance, studies indicate that introducing the CF₂H group into heterocycles can improve their efficacy against various pathogens .
- Pharmacological Potential : The incorporation of this compound into drug candidates has been linked to improved pharmacokinetic properties, such as increased lipophilicity and bioavailability. Research suggests that CF₂H can act as a hydrogen-bond donor, which may enhance interactions with biological targets .
Case Study 1: Synthesis of Difluoromethylated Heterocycles
In a study focused on the synthesis of difluoromethylated heterocycles, this compound was used as a reagent to successfully introduce the CF₂H group into various substrates. The results indicated that these modified compounds exhibited significant biological activity against specific bacterial strains.
Compound | Yield (%) | Biological Activity |
---|---|---|
Heterocycle A | 79% | Effective against E. coli |
Heterocycle B | 99% | Effective against S. aureus |
Case Study 2: Fluorination Reactions
Another research effort demonstrated the use of this compound in late-stage fluorination reactions, where it served as a key reagent for modifying existing drug scaffolds. The study highlighted the compound's ability to enhance the therapeutic profile of lead compounds by improving their metabolic stability.
Research Findings
Recent studies have revealed several important findings regarding the biological activity of this compound:
- Mechanistic Insights : The mechanism by which this compound influences biological systems involves its ability to generate reactive intermediates that can modify biomolecules such as proteins and nucleic acids .
- Toxicological Assessments : Preliminary toxicological assessments suggest that while this compound has promising applications, careful evaluation is necessary to understand its safety profile in biological systems .
Q & A
Q. Basic: What are the optimal methods for synthesizing and characterizing sodium difluoromethanesulfinate?
This compound is typically synthesized via sulfinate salt preparation, involving the reaction of difluoromethanesulfinic acid with sodium hydroxide. Purification is achieved through recrystallization in anhydrous solvents to avoid hydrolysis. Characterization requires ¹⁹F NMR (to confirm CF₂ groups) and mass spectrometry for molecular weight validation. Storage at -20°C under inert atmosphere is critical to prevent degradation .
Q. Basic: How should this compound be stored and handled to ensure stability?
To maintain stability, store the compound at -20°C in airtight, moisture-free containers under nitrogen or argon. Avoid exposure to light, moisture, or oxidizing agents. For experimental use, pre-weigh aliquots in a glovebox to minimize air exposure .
Q. Advanced: What mechanistic factors differentiate this compound from zinc difluoromethanesulfinate (DFMS) in radical generation?
Zinc difluoromethanesulfinate (DFMS) exhibits higher reactivity due to its lower oxidation potential (+1.35 V vs. SCE ) compared to sodium salts, enabling easier radical generation under mild conditions. Sodium salts may require stronger oxidants (e.g., peroxides) or photoredox catalysts. Methodologically, cyclic voltammetry should be used to compare redox profiles, while EPR spectroscopy can confirm radical intermediates .
Q. Advanced: How can this compound be optimized for visible-light-promoted difluoromethylation of heteroarenes?
Key strategies include:
- Photosensitizer selection : Use organometallic catalysts (e.g., Ir or Ru complexes) or organic dyes with redox potentials matching the sulfinate’s oxidation threshold.
- Solvent optimization : Polar aprotic solvents (e.g., MeCN) enhance solubility and electron transfer.
- Stoichiometric tuning : Excess sulfinate (4–5 equiv.) compensates for lower reactivity. Monitor progress via HPLC or ¹⁹F NMR .
Q. Advanced: How do solvent polarity and reaction conditions affect contradictory data in difluoromethylation yields?
Conflicting results often arise from solvent-dependent radical stabilization. For example, DMSO may quench radicals, while MeCN enhances their lifetime. Systematically test solvents using a Design of Experiments (DoE) approach, with kinetic studies (e.g., radical clock experiments) to quantify reaction rates. Cross-reference with computational models (DFT) to predict solvent effects .
Q. Advanced: What electrochemical methods improve this compound’s efficiency in electrocatalytic difluoromethylation?
Electrocatalysis avoids costly photocatalysts by directly generating radicals via anodic oxidation. Optimize:
- Electrode materials : Use carbon-based anodes for high surface area.
- Electrolyte composition : Tetrabutylammonium salts enhance conductivity.
- Voltage control : Apply potentials slightly above the sulfinate’s oxidation threshold (~+1.5 V ) to minimize side reactions. Validate yields against traditional peroxide-driven methods .
Q. Advanced: How can researchers address challenges in regioselective difluoromethylation using this compound?
Regioselectivity depends on substrate electronics. For electron-deficient heteroarenes:
- Additive screening : Lewis acids (e.g., Sc(OTf)₃) polarize substrates to favor radical addition at specific positions.
- Substrate pre-functionalization : Introduce directing groups (e.g., pyridine) to guide radical attack.
- Competition experiments : Compare yields with alternative radical sources (e.g., DFMS) to identify selectivity drivers .
Q. Advanced: What analytical techniques resolve discrepancies in reaction by-product identification?
Use 2D NMR (HSQC, HMBC) to trace unexpected signals and high-resolution mass spectrometry (HRMS) for precise by-product identification. For volatile by-products, GC-MS paired with isotopic labeling (e.g., ¹⁸O in peroxides) clarifies decomposition pathways .
Properties
IUPAC Name |
sodium;difluoromethanesulfinate | |
---|---|---|
Details | Computed by LexiChem 2.6.6 (PubChem release 2019.06.18) | |
Source | PubChem | |
URL | https://pubchem.ncbi.nlm.nih.gov | |
Description | Data deposited in or computed by PubChem | |
InChI |
InChI=1S/CH2F2O2S.Na/c2-1(3)6(4)5;/h1H,(H,4,5);/q;+1/p-1 | |
Details | Computed by InChI 1.0.5 (PubChem release 2019.06.18) | |
Source | PubChem | |
URL | https://pubchem.ncbi.nlm.nih.gov | |
Description | Data deposited in or computed by PubChem | |
InChI Key |
WRYSLFYACKIPNN-UHFFFAOYSA-M | |
Details | Computed by InChI 1.0.5 (PubChem release 2019.06.18) | |
Source | PubChem | |
URL | https://pubchem.ncbi.nlm.nih.gov | |
Description | Data deposited in or computed by PubChem | |
Canonical SMILES |
C(F)(F)S(=O)[O-].[Na+] | |
Details | Computed by OEChem 2.1.5 (PubChem release 2019.06.18) | |
Source | PubChem | |
URL | https://pubchem.ncbi.nlm.nih.gov | |
Description | Data deposited in or computed by PubChem | |
Molecular Formula |
CHF2NaO2S | |
Details | Computed by PubChem 2.1 (PubChem release 2019.06.18) | |
Source | PubChem | |
URL | https://pubchem.ncbi.nlm.nih.gov | |
Description | Data deposited in or computed by PubChem | |
Molecular Weight |
138.07 g/mol | |
Details | Computed by PubChem 2.1 (PubChem release 2021.05.07) | |
Source | PubChem | |
URL | https://pubchem.ncbi.nlm.nih.gov | |
Description | Data deposited in or computed by PubChem | |
CAS No. |
275818-95-6 | |
Record name | Sodium difluoromethanesulfinate | |
Source | European Chemicals Agency (ECHA) | |
URL | https://echa.europa.eu/information-on-chemicals | |
Description | The European Chemicals Agency (ECHA) is an agency of the European Union which is the driving force among regulatory authorities in implementing the EU's groundbreaking chemicals legislation for the benefit of human health and the environment as well as for innovation and competitiveness. | |
Explanation | Use of the information, documents and data from the ECHA website is subject to the terms and conditions of this Legal Notice, and subject to other binding limitations provided for under applicable law, the information, documents and data made available on the ECHA website may be reproduced, distributed and/or used, totally or in part, for non-commercial purposes provided that ECHA is acknowledged as the source: "Source: European Chemicals Agency, http://echa.europa.eu/". Such acknowledgement must be included in each copy of the material. ECHA permits and encourages organisations and individuals to create links to the ECHA website under the following cumulative conditions: Links can only be made to webpages that provide a link to the Legal Notice page. | |
Disclaimer and Information on In-Vitro Research Products
Please be aware that all articles and product information presented on BenchChem are intended solely for informational purposes. The products available for purchase on BenchChem are specifically designed for in-vitro studies, which are conducted outside of living organisms. In-vitro studies, derived from the Latin term "in glass," involve experiments performed in controlled laboratory settings using cells or tissues. It is important to note that these products are not categorized as medicines or drugs, and they have not received approval from the FDA for the prevention, treatment, or cure of any medical condition, ailment, or disease. We must emphasize that any form of bodily introduction of these products into humans or animals is strictly prohibited by law. It is essential to adhere to these guidelines to ensure compliance with legal and ethical standards in research and experimentation.