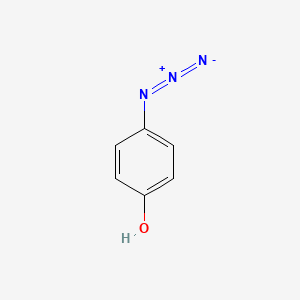
4-Azidophenol
Overview
Description
4-Azidophenol (CAS: 16331-58-9) is a para-substituted phenol derivative featuring an azide (-N₃) functional group. It is synthesized via diazotization of 4-aminophenol followed by azide substitution, yielding a dark-brown liquid with high purity (95% yield) . Key spectral data include:
- IR: Strong azide absorption at 2106 cm⁻¹ and O-H stretching at 3365 cm⁻¹ .
- NMR: Para-substitution confirmed by AA′BB′ coupling (δ 6.89–6.80 ppm in $^1$H NMR; δ 153.7 ppm for phenolic carbon in $^13$C NMR) .
Computational studies (M06-2X/6-311++G) reveal its planar geometry and electronic structure, with gas-phase acidity influenced by the electron-withdrawing azide group . The compound is widely utilized in click chemistry and polymer functionalization due to its reactive azide and phenolic hydroxyl groups .
Preparation Methods
Synthetic Routes and Reaction Conditions: 4-Azidophenol can be synthesized from 4-aminophenol through a diazotization reaction followed by azidation. The process involves the following steps :
Diazotization: 4-aminophenol is suspended in water, and concentrated hydrochloric acid is added dropwise. The reaction mixture is then cooled to 0°C, and a solution of sodium nitrite in water is added. This forms the diazonium salt.
Azidation: Sodium azide is added to the diazonium salt solution, resulting in the formation of this compound.
Industrial Production Methods: While specific industrial production methods for this compound are not widely documented, the general approach involves scaling up the laboratory synthesis process. This includes optimizing reaction conditions, such as temperature and reagent concentrations, to ensure high yield and purity.
Chemical Reactions Analysis
Types of Reactions: 4-Azidophenol undergoes various chemical reactions, including:
Substitution Reactions: this compound can react with benzyl bromide derivatives in the presence of potassium carbonate to form substituted azides.
Common Reagents and Conditions:
Photolysis: Low-temperature matrices (12–14 K) and flash vacuum pyrolysis (FVP) are commonly used conditions.
Substitution: Benzyl bromide derivatives and potassium carbonate are typical reagents.
Major Products:
Azepin-4-ones: Formed through photolysis and ring expansion.
Substituted Azides: Formed through substitution reactions with benzyl bromide derivatives.
Scientific Research Applications
4-Azidophenol has several scientific research applications, including:
Enzymatic Activity and Drug Interactions: Derivatives of this compound, such as 4-azido-2-hydroxybenzoic acid, are used to study the binding sites and enzymatic activities of specific proteins like UDP-glucuronosyltransferases.
Mitochondrial Function Studies: Derivatives like 2-azido-4-nitrophenol are used to study mitochondrial uncoupling and the binding of uncouplers to mitochondrial components.
Synthetic Chemistry: this compound is used in low-temperature matrices to synthesize novel compounds like 4H-azepin-4-ones.
Polymer Science:
Bioconjugation Techniques: The photochemical properties of compounds like 4-(6-formyl-3-azidophenoxy)butyrimidate are used to study protein interactions.
Mechanism of Action
The mechanism of action of 4-azidophenol involves its ability to undergo photolysis and substitution reactions. The azide group can be activated by light or heat, leading to the formation of reactive intermediates such as nitrenes. These intermediates can then participate in various chemical reactions, including ring expansion and substitution . The molecular targets and pathways involved depend on the specific application and the nature of the derivatives used.
Comparison with Similar Compounds
Structural Analogs: Azide-Functionalized Phenols
4-Azidobenzoic Acid
- Structure: Replaces the hydroxyl (-OH) of 4-azidophenol with a carboxylic acid (-COOH).
- Acidity: Significantly more acidic (pKa ~2–3) due to the -COOH group, compared to this compound (pKa ~8–10, estimated from gas-phase studies) .
- Applications : Used in UV-grafting of anti-fouling polymers, leveraging both azide reactivity and carboxylic acid functionality .
Ortho- and Meta-Azidophenols
- Reactivity: Positional isomerism affects electronic properties.
Substituent Effects on Acidity
The azide group exerts a strong electron-withdrawing effect, increasing phenol acidity relative to unsubstituted phenol. Computational data suggest a gas-phase acidity (ΔG°) of this compound is lower (more acidic) than phenol due to resonance and inductive effects . Comparatively:
- Phenol (C₆H₅OH): ΔG° ≈ 338 kcal/mol (reference value).
- 4-Nitrophenol: A stronger acid (pKa ~7.1) than this compound, highlighting nitro’s greater electron-withdrawing capacity.
Reactivity in Click Chemistry
This compound participates in copper-catalyzed azide-alkyne cycloaddition (CuAAC) to form 1,2,3-triazoles. Key comparisons:
- Phenyl Azide (C₆H₅N₃): Lacks the phenolic -OH, reducing hydrogen-bonding capacity and solubility in polar solvents. This compound’s -OH enhances aqueous compatibility .
- Propargyl Derivatives: In -azidophenol reacts with propargyl ethers to yield triazoles with >90% efficiency, outperforming non-phenolic azides in hydrophilic reaction media .
Bisphenol Derivatives
- 4,4'-(Propane-2,2-diyl)diphenol (Bisphenol A): Lacks azide functionality but shares phenolic -OH groups. The electron-donating isopropylidene group reduces acidity compared to this compound .
- 4,4'-Sulfonyldiphenol (Bisphenol S): Sulfone group is electron-withdrawing, increasing acidity but less reactive than azide in click chemistry .
Data Tables
Table 1: Spectral and Physical Properties of this compound and Analogs
Compound | IR (cm⁻¹) | $^1$H NMR (δ, ppm) | Acidity (ΔG°, kcal/mol) |
---|---|---|---|
This compound | 2106 (N₃), 3365 | 6.89–6.80 (AA′BB′) | Computationally lower |
Phenol | 3350 (O-H) | 6.6–7.4 (aromatic) | 338 (reference) |
4-Azidobenzoic Acid | 2100 (N₃), 1700 | 7.8–8.2 (aromatic) | Not reported |
Table 2: Reactivity in CuAAC Reactions
Azide Component | Alkyne Partner | Solvent | Yield (%) |
---|---|---|---|
This compound | Propargyl ether | H₂O/t-BuOH | >90 |
Phenyl Azide | Propargyl ether | Organic phase | 75–85 |
Biological Activity
4-Azidophenol is an organic compound with significant biological activity, characterized by its unique phenolic structure and the presence of an azide group at the para position relative to the hydroxyl group. This compound has garnered attention for its potential applications in biochemical pathways, enzyme interactions, and cellular processes.
Chemical Structure and Properties
The molecular formula of this compound is . Its structure consists of a benzene ring with a hydroxyl group (-OH) and an azide group (-N₃) attached, which imparts distinctive chemical properties that facilitate its biological interactions. The compound is known for its light sensitivity and ability to undergo photoinduced reactions, making it a valuable tool in chemical biology.
Biological Activity
1. Enzyme Interactions
This compound has been shown to interact with various enzymes, notably phenol sulfotransferase. This enzyme is crucial for drug metabolism and detoxification processes in human tissues such as the liver and brain. The azide group enables this compound to participate in bioorthogonal reactions, which are essential for labeling biomolecules in cellular studies. Research indicates that this compound can influence enzyme kinetics and modify protein structures through covalent bonding mechanisms, thereby affecting biological functions significantly .
2. Cellular Signaling and Gene Expression
Studies have indicated that this compound may modulate cellular signaling pathways and gene expression. Its ability to affect these pathways suggests potential therapeutic applications in various diseases, including cancer. For instance, it has been found to alter cell proliferation rates and influence apoptosis in certain cell lines .
Case Studies
Several studies have highlighted the biological effects of this compound:
- Study on Enzyme Inhibition : A focused compound collection involving this compound derivatives was screened for inhibition of macrophage migration inhibitory factor (MIF) tautomerase activity. The results demonstrated that certain derivatives exhibited significant inhibitory effects, suggesting a structure-activity relationship that could be exploited for therapeutic purposes .
- Photochemical Applications : Research has explored the use of this compound in photochemical reactions, where UV light exposure leads to the formation of reactive nitrene species. These species can facilitate crosslinking within proteins, altering their functional properties and potentially enhancing their utility in biomedical applications .
Comparative Analysis
To better understand the unique properties of this compound, it is useful to compare it with structurally similar compounds:
Compound Name | Structural Characteristics | Unique Features |
---|---|---|
3-Azidophenol | Azide group at the meta position | Different electronic properties due to substitution pattern |
4-Azido-2-nitrophenol | Nitro group at the ortho position | Increased reactivity compared to this compound |
2,6-Dichloro-4-azidophenol | Two chlorine substituents | Enhanced stability due to halogen effects |
5-Azido-8-hydroxyquinoline | Heterocyclic structure with an azide group | Unique reactivity due to heteroatom presence |
Synthesis Methods
The synthesis of this compound typically involves two main steps:
- Preparation of Phenolic Compound : Starting from readily available phenolic precursors.
- Azidation Reaction : Introduction of the azide group through nucleophilic substitution or other methods.
Industrial synthesis may optimize these laboratory methods to enhance yield and purity .
Q & A
Basic Research Questions
Q. How can 4-azidophenol be synthesized with high purity for spectroscopic characterization?
- Methodology : Use nucleophilic aromatic substitution by reacting 4-fluorophenol with sodium azide in dimethyl sulfoxide (DMSO) at 80–100°C for 12–24 hours. Monitor reaction progress via thin-layer chromatography (TLC) with ethyl acetate/hexane (1:4) as the mobile phase. Purify via column chromatography (silica gel, gradient elution) and confirm purity using -NMR (absence of azide proton signal at ~3.5 ppm) and mass spectrometry (m/z 151.04 [M+H]) .
- Key Challenge : Avoid side reactions (e.g., phenol oxidation) by maintaining an inert atmosphere (N) and controlling reaction temperature.
Q. What spectroscopic techniques are optimal for characterizing this compound’s structure and stability?
- Methodology :
- FT-IR : Confirm azide (-N) stretch at 2100–2150 cm and phenolic O-H stretch at 3200–3600 cm .
- UV-Vis : Monitor photodegradation under UV light (λ = 254 nm) to assess stability; track absorbance decay at λ ~270 nm .
- Thermogravimetric Analysis (TGA) : Determine decomposition temperature (T) under nitrogen to evaluate thermal stability .
Advanced Research Questions
Q. How do substituent effects influence the electronic properties of this compound in gas-phase studies?
- Methodology : Perform density functional theory (DFT) calculations (e.g., M06-2X/6-311++G** basis set) to analyze charge distribution, dipole moments, and gas-phase acidity (ΔG) . Compare with experimental gas-phase acidity measurements using mass spectrometry-based equilibrium methods.
- Data Contradictions : Computational models may overestimate electron-withdrawing effects of the azide group compared to experimental data due to solvation neglect. Validate results with solvent-phase NMR chemical shifts .
Q. What experimental designs mitigate risks when studying this compound’s photolytic decomposition?
- Methodology :
- Light Sources : Use monochromatic UV lamps (e.g., 254 nm) to isolate photolytic pathways.
- Quenching Agents : Add tert-butanol to scavenge hydroxyl radicals and differentiate between direct photolysis and radical-mediated degradation .
- Analytical Validation : Employ LC-MS/MS to identify intermediates (e.g., nitroso or amine derivatives) and quantify degradation kinetics .
Q. How can computational and experimental data resolve contradictions in this compound’s reactivity with biomolecules?
- Methodology :
- Molecular Dynamics (MD) Simulations : Model interactions with cysteine residues to predict nucleophilic attack sites.
- Experimental Validation : Use stopped-flow kinetics to measure reaction rates with glutathione (GSH) at pH 7.4, comparing computational activation energies (E) with Arrhenius-derived values .
- Challenge : Discrepancies may arise from solvent effects (e.g., aqueous vs. gas-phase simulations).
Q. Safety and Best Practices
Q. What safety protocols are critical for handling this compound in laboratory settings?
- Guidelines :
- Personal Protective Equipment (PPE) : Nitrile gloves, lab coat, and UV-protective goggles (azides are light-sensitive and potentially explosive) .
- Storage : Keep in amber vials at –20°C under nitrogen to prevent azide decomposition.
- Waste Disposal : Neutralize with sodium nitrite/hydrochloric acid before disposal to eliminate explosive hazards .
Q. Data Interpretation and Reporting
Q. How should researchers address discrepancies between theoretical and experimental vibrational spectra of this compound?
- Methodology :
- Anharmonic Corrections : Apply DFT-level anharmonic frequency calculations to better match experimental IR peaks (e.g., azide stretching modes) .
- Cross-Validation : Compare results with Raman spectroscopy to confirm assignments and rule out solvent artifacts .
Q. What strategies ensure reproducibility in studies comparing this compound with structural analogs (e.g., 4-aminophenol)?
- Methodology :
Properties
IUPAC Name |
4-azidophenol | |
---|---|---|
Details | Computed by Lexichem TK 2.7.0 (PubChem release 2021.05.07) | |
Source | PubChem | |
URL | https://pubchem.ncbi.nlm.nih.gov | |
Description | Data deposited in or computed by PubChem | |
InChI |
InChI=1S/C6H5N3O/c7-9-8-5-1-3-6(10)4-2-5/h1-4,10H | |
Details | Computed by InChI 1.0.6 (PubChem release 2021.05.07) | |
Source | PubChem | |
URL | https://pubchem.ncbi.nlm.nih.gov | |
Description | Data deposited in or computed by PubChem | |
InChI Key |
ABSZNIJDTSIVHN-UHFFFAOYSA-N | |
Details | Computed by InChI 1.0.6 (PubChem release 2021.05.07) | |
Source | PubChem | |
URL | https://pubchem.ncbi.nlm.nih.gov | |
Description | Data deposited in or computed by PubChem | |
Canonical SMILES |
C1=CC(=CC=C1N=[N+]=[N-])O | |
Details | Computed by OEChem 2.3.0 (PubChem release 2021.05.07) | |
Source | PubChem | |
URL | https://pubchem.ncbi.nlm.nih.gov | |
Description | Data deposited in or computed by PubChem | |
Molecular Formula |
C6H5N3O | |
Details | Computed by PubChem 2.1 (PubChem release 2021.05.07) | |
Source | PubChem | |
URL | https://pubchem.ncbi.nlm.nih.gov | |
Description | Data deposited in or computed by PubChem | |
Molecular Weight |
135.12 g/mol | |
Details | Computed by PubChem 2.1 (PubChem release 2021.05.07) | |
Source | PubChem | |
URL | https://pubchem.ncbi.nlm.nih.gov | |
Description | Data deposited in or computed by PubChem | |
Synthesis routes and methods
Procedure details
Disclaimer and Information on In-Vitro Research Products
Please be aware that all articles and product information presented on BenchChem are intended solely for informational purposes. The products available for purchase on BenchChem are specifically designed for in-vitro studies, which are conducted outside of living organisms. In-vitro studies, derived from the Latin term "in glass," involve experiments performed in controlled laboratory settings using cells or tissues. It is important to note that these products are not categorized as medicines or drugs, and they have not received approval from the FDA for the prevention, treatment, or cure of any medical condition, ailment, or disease. We must emphasize that any form of bodily introduction of these products into humans or animals is strictly prohibited by law. It is essential to adhere to these guidelines to ensure compliance with legal and ethical standards in research and experimentation.