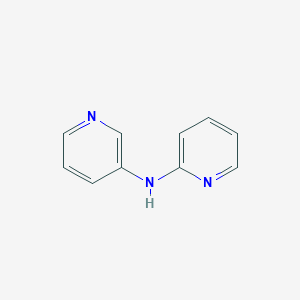
N-(Pyridin-3-yl)pyridin-2-amine
Overview
Description
N-(Pyridin-3-yl)pyridin-2-amine is a bicyclic aromatic amine featuring two pyridine rings connected via an amine linkage between the 3-position of one pyridine and the 2-position of the other. This structural motif is pivotal in medicinal chemistry due to its ability to engage in hydrogen bonding, π-π stacking, and metal coordination, which underpin its pharmacological and material science applications.
Preparation Methods
Synthetic Routes and Reaction Conditions
N-(Pyridin-3-yl)pyridin-2-amine can be synthesized through several methods. One common approach involves the reaction of 2-aminopyridine with α-bromoketones under specific conditions. For instance, the compound can be formed in toluene via C–C bond cleavage promoted by iodine (I2) and tert-butyl hydroperoxide (TBHP) under mild and metal-free conditions . Another method involves the use of nitrostyrenes and 2-aminopyridines via the corresponding N-(pyridin-2-yl)iminonitriles as intermediates .
Industrial Production Methods
Industrial production methods for this compound typically involve large-scale synthesis using optimized reaction conditions to ensure high yield and purity. These methods often employ continuous flow reactors and automated systems to control reaction parameters precisely.
Chemical Reactions Analysis
Types of Reactions
N-(Pyridin-3-yl)pyridin-2-amine undergoes various chemical reactions, including:
Oxidation: The compound can be oxidized using oxidizing agents such as hydrogen peroxide (H2O2) or potassium permanganate (KMnO4).
Reduction: Reduction reactions can be carried out using reducing agents like sodium borohydride (NaBH4) or lithium aluminum hydride (LiAlH4).
Substitution: The compound can participate in substitution reactions, where functional groups on the pyridine rings are replaced with other groups.
Common Reagents and Conditions
Common reagents used in these reactions include iodine (I2), tert-butyl hydroperoxide (TBHP), and various oxidizing and reducing agents. Reaction conditions typically involve controlled temperatures, solvents like toluene or ethyl acetate, and catalysts to facilitate the reactions.
Major Products Formed
The major products formed from these reactions depend on the specific reaction conditions and reagents used. For example, oxidation reactions may yield pyridine N-oxides, while reduction reactions can produce amine derivatives.
Scientific Research Applications
N-(2-Methyl-5-nitrophenyl)-4-(pyridin-3-yl)pyrimidin-2-amine is a chemical compound that belongs to the class of 2-aminopyrimidine derivatives and serves as a precursor in the synthesis of active pharmaceutical ingredients (APIs) . This compound is primarily used in the production of imatinib, an antineoplastic drug, and has shown potential in creating substances with antibacterial and antifungal activities .
Synthesis and Production
The preparation of N-(2-methyl-5-nitrophenyl)-4-(pyridin-3-yl)pyrimidin-2-amine involves a method where 3-(dimethylamino)-1-(3-pyridyl)prop-2-en-1-one is mixed with 1-(2-methyl-5-nitrophenyl)-guanidine nitrate and an alkali hydroxide, such as sodium hydroxide, in a solvent like dimethyl sulfoxide (DMSO) . This mixture undergoes a coupling reaction under specific conditions, including a pressure of 1 to 50 MPa and a rotating microwave field with a controlled absorbed microwave power volumetric density . The reaction is conducted at a temperature between 300 and 773K, ensuring that the volumetric density of microwave power absorbed by the reaction system is controlled during the synthesis .
Role as a Precursor
N-(2-Methyl-5-nitrophenyl)-4-(pyridin-3-yl)pyrimidin-2-amine acts as a crucial precursor in the synthesis of several active pharmaceutical ingredients .
Imatinib Mesylate
It is used in the production of imatinib mesylate, a tyrosine kinase inhibitor . Imatinib mesylate is effective in treating chronic myelogenous leukemia, acute lymphoblastic leukemia, mesenchymal neoplasms of alimentary tract cells, and skin fibrosarcomata .
Antibacterial and Antifungal Agents
The compound is also utilized to produce 4-methyl-3-(4-pyridin-3-yl-pyrimidin-2-yl-amine)-phenylamine-2,5-dichloro-4-hydroxy-3,6-dioxo-cyclohexa-1,4-dienol (chloranilic acid N-(5-nitro-2-methylphenyl)-4-(3-pyridyl)-2-pyrimidineamine salt), which exhibits antibacterial and antifungal activities in in vitro tests .
Nilotinib
N-(2-Methyl-5-nitrophenyl)-4-(pyridin-3-yl)pyrimidin-2-amine is also a precursor in the synthesis of nilotinib, another tyrosine kinase inhibitor . Nilotinib selectively inhibits proliferation and induces apoptosis in cell lines and primary cells of Philadelphia chromosome leukemia in patients with chronic lymphoblastic leukemia .
Pyridin-2-amine derivatives
N-aryl-4-(pyridin-3-yl)pyrimidin-2-amine derivatives can be synthesized from corresponding amines using Buchwald-Hartwig amination . Pyridin-2-amines are used as starting material for the production of fused heterocycles, including imidazo-derivatives, that possess biological activities, such as antiviral and immunosuppressive agents . 3-amino-imidazo[1,2-a]pyridines have been identified as inhibitors of Mycobacterium tuberculosis glutamine synthetase, and 6-aminopyridin-3-ol has been used in the synthesis of new antibiotics .
Applications in Drug Design
Mechanism of Action
The mechanism of action of N-(Pyridin-3-yl)pyridin-2-amine involves its interaction with specific molecular targets, such as enzymes or receptors. The compound can bind to active sites or allosteric sites, modulating the activity of the target molecule. This interaction can lead to various biological effects, including inhibition or activation of enzymatic activity and modulation of signal transduction pathways .
Comparison with Similar Compounds
Structural and Functional Analogues: Comparative Analysis
Positional Isomers: N-(Pyridin-2-yl)pyridin-2-amine (2,2'-Dipyridylamine)
- Structure : The positional isomer, 2,2'-dipyridylamine (CAS 1202-34-2), differs in the connectivity of the pyridine rings (2-2' linkage vs. 3-2 in the target compound).
- Properties : The altered linkage reduces steric hindrance, enhancing its utility as a bidentate ligand in coordination chemistry. For example, Co(II) and Cu(II) complexes of similar ligands (e.g., N-((1H-pyrrol-2-yl)methylene)-6-methoxypyridin-3-amine) exhibit distinct spectroscopic and magnetic behaviors, suggesting that the 3-2 linkage in the target compound may offer unique metal-binding selectivity .
Schiff Base Derivatives of 2-Aminopyridine
- Examples :
- N-(2-Hydroxybenzylidene)pyridin-2-amine (I)
- N-(5-Nitro-2-hydroxybenzylidene)pyridin-2-amine (II)
- Synthesis: Prepared via condensation of 2-aminopyridine with substituted benzaldehydes.
- Bioactivity: These derivatives exhibit moderate antimicrobial activity against Staphylococcus aureus and Escherichia coli, with activity influenced by substituents. For instance, the nitro group in compound II enhances electron-withdrawing effects, improving solubility in polar solvents like DMF and enabling keto-enol tautomerism, which may enhance membrane penetration .
- Comparison : The absence of a Schiff base linkage in N-(Pyridin-3-yl)pyridin-2-amine likely reduces its tautomeric versatility but may improve metabolic stability in vivo.
Anticancer Agents with Pyridinamine Motifs
Pexidartinib (PLX3397)
- Structure : Contains a pyridinamine core (N-[[6-(trifluoromethyl)pyridin-3-yl]methyl]pyridin-2-amine) with a pyrrolopyridine substituent.
- Activity: Potent inhibitor of CSF1R and KIT tyrosine kinases, approved for tenosynovial giant cell tumor. Its trifluoromethyl group enhances lipophilicity and target binding affinity.
- Metabolism : Undergoes hepatic CYP3A4-mediated oxidation, forming reactive metabolites that may contribute to hepatotoxicity. This highlights the importance of substituent choice in balancing efficacy and safety .
FLT3/BCR-ABL Inhibitors
- Example : N-(3-Methoxyphenyl)-6-(7-(1-methyl-1H-pyrazol-4-yl)imidazo[1,2-a]pyridin-3-yl)pyridin-2-amine.
- Activity : Inhibits FLT3-ITD and BCR-ABL pathways (IC50 < 10 nM) and overcomes resistance mutations (D835Y, F691L). The imidazopyridine moiety enhances kinase selectivity compared to simpler pyridinamine scaffolds .
CYP51 Inhibitors for Chagas Disease
- Examples: (S)-(4-Chlorophenyl)-1-(4-(4-(trifluoromethyl)phenyl)-piperazin-1-yl)-2-(pyridin-3-yl)ethanone (UDO) N-[4-(Trifluoromethyl)phenyl]-N-[1-[5-(trifluoromethyl)-2-pyridyl]-4-piperidyl]pyridin-3-amine (UDD)
- Activity: Non-azole CYP51 inhibitors with efficacy against Trypanosoma cruzi comparable to posaconazole. The trifluoromethyl groups enhance binding to the enzyme's hydrophobic active site, a feature absent in this compound .
Biological Activity
N-(Pyridin-3-yl)pyridin-2-amine is a compound of significant interest in medicinal chemistry due to its diverse biological activities. This article synthesizes findings from various studies that highlight its potential as an anticancer agent, its antibacterial and antifungal properties, and its role in inhibiting specific biological targets.
Synthesis and Structure
This compound and its derivatives have been synthesized using various methods, including the Buchwald-Hartwig amination technique. This method allows for the formation of new N-aryl derivatives with moderate to good yields (27% to 82%) . The structural characterization of these compounds is typically confirmed through techniques such as FT-NMR and FT-IR spectroscopy.
Anticancer Activity
A pivotal study evaluated a series of pyridin-3-yl pyrimidines, including this compound derivatives, for their Bcr-Abl inhibitory and anticancer activities. Notably, certain compounds exhibited potent inhibition against Bcr-Abl, suggesting their potential as therapeutic agents for leukemia .
Case Study: A549 Cell Line
In a comparative analysis, the anticancer effects of these derivatives were found to surpass those of imatinib, a widely used cancer drug. The derivatives demonstrated significant cytotoxicity against the A549 human lung cancer cell line, indicating their potential in treating lung cancer .
Antibacterial and Antifungal Properties
Research has also focused on the antibacterial and antifungal activities of this compound derivatives. These compounds have shown effectiveness against various bacterial strains, including Gram-positive and Gram-negative species, as well as fungal pathogens. The biological activity was often superior to that of established commercial antibiotics .
Table 1: Summary of Biological Activities
Activity | Target Organisms | Results |
---|---|---|
Anticancer | A549 (lung cancer) | Higher efficacy than imatinib |
Antibacterial | Gram-positive and Gram-negative | Superior activity compared to commercial drugs |
Antifungal | Various fungal species | Effective against specialized aquatic bacteria |
Antioxidant | General antioxidant assays | IC50 values comparable to ascorbic acid |
The mechanism by which this compound exerts its biological effects is multifaceted. Molecular docking studies suggest that these compounds interact with specific targets such as receptor tyrosine kinases and other enzymes involved in cancer progression .
In Silico Studies
In silico investigations have highlighted the binding affinities of these compounds to various molecular targets, indicating a promising ADME-Tox profile that supports further clinical exploration .
Q & A
Basic Research Questions
Q. What are the optimal synthetic routes for N-(Pyridin-3-yl)pyridin-2-amine, and what methodological considerations ensure reproducibility?
The synthesis of N-(Pyridin-3-yl)pyridin-2-amine typically involves nucleophilic aromatic substitution (NAS) or palladium-catalyzed coupling reactions. For example, microwave-assisted protocols (50–120°C, 15–30 minutes) under inert atmospheres can enhance reaction efficiency and yield, as demonstrated in the synthesis of related pyridin-3-amine derivatives . Key considerations include:
- Catalyst selection : Pd(OAc)₂ or Pd(dba)₂ with ligands like XPhos for cross-coupling.
- Solvent optimization : Use polar aprotic solvents (e.g., DMF or DMSO) to stabilize intermediates.
- Purification : Column chromatography (silica gel, hexane/EtOAc gradient) or recrystallization from ethanol/water mixtures .
Q. What spectroscopic techniques are most effective for characterizing this compound, and how should data be interpreted?
Combined use of ¹H/¹³C NMR and FT-IR spectroscopy is critical:
- NMR : Look for aromatic proton signals in δ 6.8–8.5 ppm (pyridine ring protons) and amine protons (δ 4.5–5.5 ppm, broad singlet). Coupling constants (J = 2–5 Hz) confirm substitution patterns.
- IR : Stretching bands at ~3400 cm⁻¹ (N-H) and ~1600 cm⁻¹ (C=N/C=C) validate the amine and aromatic moieties. Compare with literature spectra of analogous compounds to resolve ambiguities .
Q. How can researchers mitigate common byproducts during synthesis?
Byproducts often arise from incomplete substitution or oxidation. Strategies include:
- Temperature control : Maintain reaction temperatures below 150°C to avoid decomposition.
- Protecting groups : Use acetyl or tert-butoxycarbonyl (Boc) groups to shield reactive amines during coupling steps .
- Real-time monitoring : TLC (Rf = 0.3–0.5 in EtOAc/hexane) or LC-MS to track reaction progress .
Advanced Research Questions
Q. How can density functional theory (DFT) predict the electronic properties of this compound, and what functional parameters are optimal?
Hybrid functionals (e.g., B3LYP) with 6-311++G(d,p) basis sets accurately model the compound’s HOMO-LUMO gaps and charge distribution. Key steps:
Geometry optimization : Use Becke’s three-parameter exchange-correlation functional for thermochemical accuracy .
Solvent effects : Include implicit solvent models (e.g., PCM for DMSO) to simulate experimental conditions.
Validation : Compare computed vibrational frequencies (IR) and NMR chemical shifts with experimental data to refine parameters .
Q. What crystallographic strategies resolve structural ambiguities in this compound derivatives?
Single-crystal X-ray diffraction (SCXRD) with SHELXL refinement is gold-standard:
- Data collection : Use Mo-Kα radiation (λ = 0.71073 Å) at 100 K to minimize thermal motion.
- Refinement : Apply anisotropic displacement parameters for non-H atoms and riding models for H atoms.
- Validation : Check R1/wR2 values (< 0.05) and Flack parameter for chiral centers. Recent SHELX updates enable handling of twinned or high-disorder crystals .
Q. How do structural modifications of this compound influence bioactivity in kinase inhibition assays?
Structure-activity relationship (SAR) studies require:
- Substitution patterns : Introduce electron-withdrawing groups (e.g., -NO₂, -CF₃) at the pyridine C4 position to enhance binding to ATP pockets in kinases like Akt1.
- Functional assays : Measure IC₅₀ values using fluorescence polarization (FP) or TR-FRET assays with recombinant kinases.
- In silico docking : Use AutoDock Vina to predict binding modes, guided by co-crystal structures of related inhibitors (e.g., PDB: 4EKL) .
Q. How should researchers address contradictions between computational predictions and experimental data (e.g., reactivity or stability)?
Methodological reconciliation involves:
Error analysis : Check DFT convergence criteria (energy cutoffs < 1e⁻⁶ Hartree) and basis set superposition errors (BSSE).
Experimental replication : Repeat syntheses under rigorously anhydrous/anaerobic conditions to exclude environmental artifacts.
Multivariate statistics : Apply principal component analysis (PCA) to NMR or HPLC datasets to identify outlier reactions .
Properties
IUPAC Name |
N-pyridin-3-ylpyridin-2-amine | |
---|---|---|
Details | Computed by LexiChem 2.6.6 (PubChem release 2019.06.18) | |
Source | PubChem | |
URL | https://pubchem.ncbi.nlm.nih.gov | |
Description | Data deposited in or computed by PubChem | |
InChI |
InChI=1S/C10H9N3/c1-2-7-12-10(5-1)13-9-4-3-6-11-8-9/h1-8H,(H,12,13) | |
Details | Computed by InChI 1.0.5 (PubChem release 2019.06.18) | |
Source | PubChem | |
URL | https://pubchem.ncbi.nlm.nih.gov | |
Description | Data deposited in or computed by PubChem | |
InChI Key |
JDOUVZLLXQKXPQ-UHFFFAOYSA-N | |
Details | Computed by InChI 1.0.5 (PubChem release 2019.06.18) | |
Source | PubChem | |
URL | https://pubchem.ncbi.nlm.nih.gov | |
Description | Data deposited in or computed by PubChem | |
Canonical SMILES |
C1=CC=NC(=C1)NC2=CN=CC=C2 | |
Details | Computed by OEChem 2.1.5 (PubChem release 2019.06.18) | |
Source | PubChem | |
URL | https://pubchem.ncbi.nlm.nih.gov | |
Description | Data deposited in or computed by PubChem | |
Molecular Formula |
C10H9N3 | |
Details | Computed by PubChem 2.1 (PubChem release 2019.06.18) | |
Source | PubChem | |
URL | https://pubchem.ncbi.nlm.nih.gov | |
Description | Data deposited in or computed by PubChem | |
Molecular Weight |
171.20 g/mol | |
Details | Computed by PubChem 2.1 (PubChem release 2021.05.07) | |
Source | PubChem | |
URL | https://pubchem.ncbi.nlm.nih.gov | |
Description | Data deposited in or computed by PubChem | |
Synthesis routes and methods I
Procedure details
Synthesis routes and methods II
Procedure details
Disclaimer and Information on In-Vitro Research Products
Please be aware that all articles and product information presented on BenchChem are intended solely for informational purposes. The products available for purchase on BenchChem are specifically designed for in-vitro studies, which are conducted outside of living organisms. In-vitro studies, derived from the Latin term "in glass," involve experiments performed in controlled laboratory settings using cells or tissues. It is important to note that these products are not categorized as medicines or drugs, and they have not received approval from the FDA for the prevention, treatment, or cure of any medical condition, ailment, or disease. We must emphasize that any form of bodily introduction of these products into humans or animals is strictly prohibited by law. It is essential to adhere to these guidelines to ensure compliance with legal and ethical standards in research and experimentation.