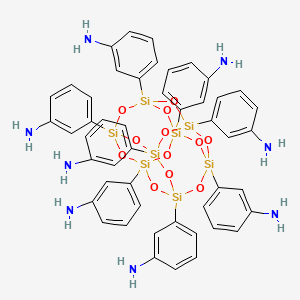
Octa(aminophenyl)-t8-silsesquioxane
- Click on QUICK INQUIRY to receive a quote from our team of experts.
- With the quality product at a COMPETITIVE price, you can focus more on your research.
Overview
Description
Octa(aminophenyl)-t8-silsesquioxane: is a type of polyhedral oligomeric silsesquioxane (POSS) compound. It is characterized by a cubic structure with eight phenyl groups, each functionalized with an amino group. This compound is known for its unique combination of organic and inorganic properties, making it a versatile material in various scientific and industrial applications .
Mechanism of Action
Target of Action
Octa(aminophenyl)-T8-silesquioxane (OAPS) primarily targets organic polymeric resins . The compound’s eight aniline-like amino groups, one on each corner silicon atom, can form chemical bonds or hydrogen bonds with appropriate matrix polymers or resins . This interaction enhances the properties of the resulting hybrid materials .
Mode of Action
OAPS interacts with its targets through chemical bonding. The amino functional groups of OAPS can form chemical bonds or hydrogen bonds with appropriate matrix polymers or resins . This interaction leads to the formation of hybrid nanocomposites . The compound’s mode of action is primarily through this bonding, which allows it to enhance the properties of the materials it is incorporated into .
Biochemical Pathways
The compound’s interaction with organic polymeric resins leads to changes in the thermal and mechanical properties of the resulting materials .
Pharmacokinetics
It is known that the compound exhibits good compatibility with diglycidyl ether of bisphenol-a (dgeba) epoxy resin at the molecular level, forming a transparent dgeba/oaps resin . This suggests that the compound may have favorable distribution properties when used in the synthesis of such materials .
Result of Action
The molecular and cellular effects of OAPS’s action primarily involve changes in the properties of the materials it is incorporated into. For instance, the introduction of OAPS into an epoxy system results in low mass loss rate and high char yield . Its initial decomposition temperature seems to be lowered . The compound’s action also leads to the formation of a transparent DGEBA/OAPS resin .
Action Environment
Environmental factors can influence the action, efficacy, and stability of OAPS. For example, the curing reaction of the DGEBA/OAPS prepolymer can occur under low temperatures compared with DGEBA/DDS . Furthermore, the compound shows larger angles with water than the DGEBA/DDS resin, indicating its hydrophobic nature . This suggests that the compound’s action and efficacy may be influenced by factors such as temperature and the presence of water .
Preparation Methods
Synthetic Routes and Reaction Conditions: The synthesis of Octa(aminophenyl)-t8-silsesquioxane typically involves a two-step process. The first step is the nitration of octaphenyl silsesquioxane to form octa(nitrophenyl)silsesquioxane. This is followed by the reduction of the nitro groups to amino groups using hydrazine hydrate in the presence of a catalyst such as Iron(III)Chloride . The reaction conditions are carefully controlled to avoid over-nitration and ensure high yield.
Industrial Production Methods: In industrial settings, the production of this compound follows similar synthetic routes but on a larger scale. The use of continuous flow reactors and advanced purification techniques ensures the consistent quality and high purity of the final product .
Chemical Reactions Analysis
Types of Reactions: Octa(aminophenyl)-t8-silsesquioxane undergoes various chemical reactions, including:
Oxidation: The amino groups can be oxidized to nitro groups under specific conditions.
Reduction: The nitro groups can be reduced back to amino groups using reducing agents like hydrazine hydrate.
Substitution: The phenyl groups can undergo substitution reactions to introduce different functional groups.
Common Reagents and Conditions:
Oxidation: Nitric acid is commonly used for the nitration of phenyl groups.
Reduction: Hydrazine hydrate in the presence of Iron(III)Chloride catalyst is used for the reduction of nitro groups.
Substitution: Various reagents can be used depending on the desired functional group to be introduced.
Major Products:
Oxidation: Octa(nitrophenyl)silsesquioxane.
Reduction: this compound.
Substitution: Functionalized derivatives of this compound.
Scientific Research Applications
Chemistry: Octa(aminophenyl)-t8-silsesquioxane is used as a building block for the synthesis of hybrid nanocomposites. Its unique structure allows for the formation of materials with enhanced thermal and mechanical properties .
Biology and Medicine: In biological research, this compound is explored for its potential use in drug delivery systems due to its ability to form stable complexes with various drugs .
Industry: In industrial applications, this compound is used to improve the properties of polymeric materials. It enhances the thermal stability, mechanical strength, and oxidation resistance of polymers .
Comparison with Similar Compounds
Octaphenylsilsesquioxane: Lacks the amino functional groups, making it less reactive compared to Octa(aminophenyl)-t8-silsesquioxane.
Octa(nitrophenyl)silsesquioxane: Contains nitro groups instead of amino groups, which can be reduced to form this compound.
Uniqueness: this compound is unique due to its eight amino-functionalized phenyl groups, which provide multiple sites for chemical reactions and interactions. This makes it a versatile compound for various applications in chemistry, biology, and industry .
Properties
IUPAC Name |
4-[3,5,7,9,11,13,15-heptakis(4-aminophenyl)-2,4,6,8,10,12,14,16,17,18,19,20-dodecaoxa-1,3,5,7,9,11,13,15-octasilapentacyclo[9.5.1.13,9.15,15.17,13]icosan-1-yl]aniline |
Source
|
---|---|---|
Details | Computed by Lexichem TK 2.7.0 (PubChem release 2021.05.07) | |
Source | PubChem | |
URL | https://pubchem.ncbi.nlm.nih.gov | |
Description | Data deposited in or computed by PubChem | |
InChI |
InChI=1S/C48H48N8O12Si8/c49-33-1-17-41(18-2-33)69-57-70(42-19-3-34(50)4-20-42)60-73(45-25-9-37(53)10-26-45)62-71(58-69,43-21-5-35(51)6-22-43)64-75(47-29-13-39(55)14-30-47)65-72(59-69,44-23-7-36(52)8-24-44)63-74(61-70,46-27-11-38(54)12-28-46)67-76(66-73,68-75)48-31-15-40(56)16-32-48/h1-32H,49-56H2 |
Source
|
Details | Computed by InChI 1.0.6 (PubChem release 2021.05.07) | |
Source | PubChem | |
URL | https://pubchem.ncbi.nlm.nih.gov | |
Description | Data deposited in or computed by PubChem | |
InChI Key |
POCWMDTVORZLKC-UHFFFAOYSA-N |
Source
|
Details | Computed by InChI 1.0.6 (PubChem release 2021.05.07) | |
Source | PubChem | |
URL | https://pubchem.ncbi.nlm.nih.gov | |
Description | Data deposited in or computed by PubChem | |
Canonical SMILES |
C1=CC(=CC=C1N)[Si]23O[Si]4(O[Si]5(O[Si](O2)(O[Si]6(O[Si](O3)(O[Si](O4)(O[Si](O5)(O6)C7=CC=C(C=C7)N)C8=CC=C(C=C8)N)C9=CC=C(C=C9)N)C1=CC=C(C=C1)N)C1=CC=C(C=C1)N)C1=CC=C(C=C1)N)C1=CC=C(C=C1)N |
Source
|
Details | Computed by OEChem 2.3.0 (PubChem release 2021.05.07) | |
Source | PubChem | |
URL | https://pubchem.ncbi.nlm.nih.gov | |
Description | Data deposited in or computed by PubChem | |
Molecular Formula |
C48H48N8O12Si8 |
Source
|
Details | Computed by PubChem 2.1 (PubChem release 2021.05.07) | |
Source | PubChem | |
URL | https://pubchem.ncbi.nlm.nih.gov | |
Description | Data deposited in or computed by PubChem | |
Molecular Weight |
1153.6 g/mol |
Source
|
Details | Computed by PubChem 2.1 (PubChem release 2021.05.07) | |
Source | PubChem | |
URL | https://pubchem.ncbi.nlm.nih.gov | |
Description | Data deposited in or computed by PubChem | |
Disclaimer and Information on In-Vitro Research Products
Please be aware that all articles and product information presented on BenchChem are intended solely for informational purposes. The products available for purchase on BenchChem are specifically designed for in-vitro studies, which are conducted outside of living organisms. In-vitro studies, derived from the Latin term "in glass," involve experiments performed in controlled laboratory settings using cells or tissues. It is important to note that these products are not categorized as medicines or drugs, and they have not received approval from the FDA for the prevention, treatment, or cure of any medical condition, ailment, or disease. We must emphasize that any form of bodily introduction of these products into humans or animals is strictly prohibited by law. It is essential to adhere to these guidelines to ensure compliance with legal and ethical standards in research and experimentation.